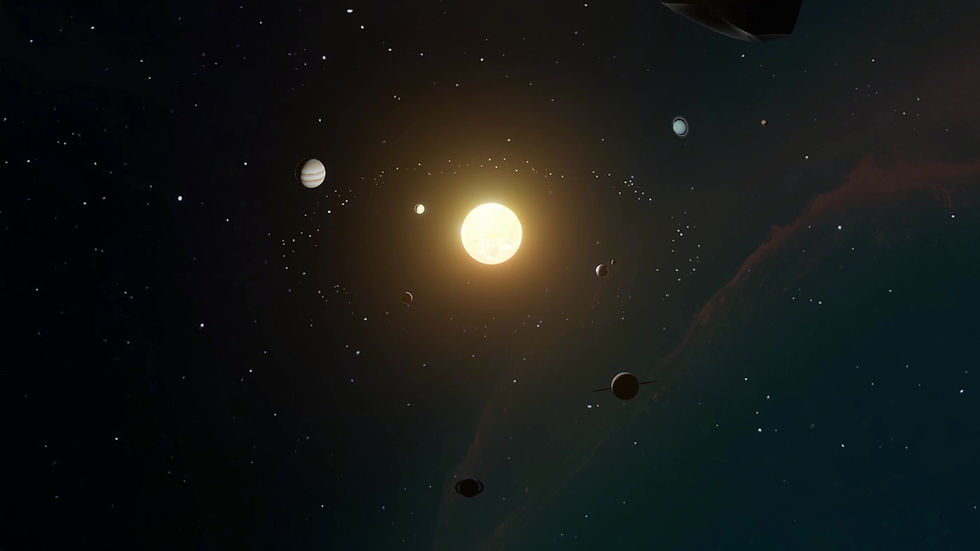
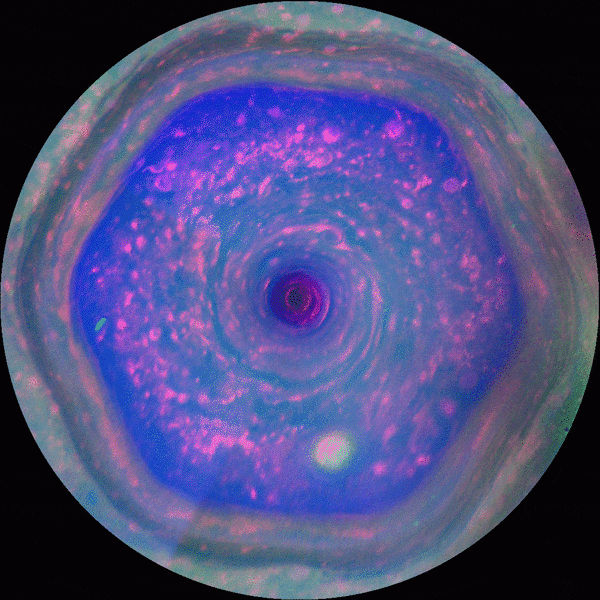
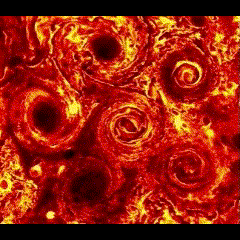
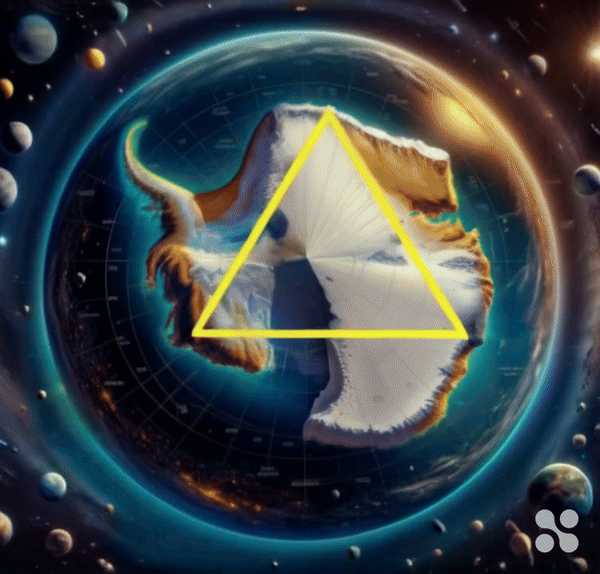
Hexagon
6th Planet
Pentagon/Octagon
5th/8 Planets
Triangle
3rd Planet
The Primer: Polygonal Alien Signatures on Jupiter & Saturn
Table of Contents
-
Geometric Patterns on Jupiter and Saturn
-
Cyclones at Jupiter's Poles
-
Saturn's Hexagonal Storm
-
Theoretical Models and Stability Mechanisms
-
-
Historical Perspectives on Alien Communication
-
Gauss's Triangle Proposal
-
Modern Interpretations
-
-
-
Deciphering the Patterns
-
Implications for Alien Communication
-
-
Building the Primer Beacon on Earth
-
Design and Engineering
-
Technological Requirements
-
-
Antarctic Colonization and $PRIM Currency
-
Incentives and Economics
-
Challenges and Solutions
-
-
Electromagnetic Considerations
-
Magnetic Field Effects on Human Health
-
Energy Harvesting Techniques
-
-
Tesla's Wireless Energy Transmission
-
Historical Background
-
Modern Applications
-
-
Extraterrestrial Life and Genetic Parallels
-
Historical Encounters
-
Biological Implications
-
-
-
Current Status
-
Future Prospects
-
-
New Model of Planetary Formation
-
Decay Model of Planetary Formation
-
New Insights from Modern Astronomy
-
-
Biblical Prophecies and Modern Science
-
Interpretations of Ancient Texts
-
Future Predictions
-
-
-
Ice Removal from South Pole
-
Polar Generator
-
- Recommendations
- Conclusion
-
Summary of Key Points
-
Future Research Directions
-
Section 1: Introduction
The quest to understand our place in the universe has driven human curiosity and scientific inquiry for centuries. Humanity has always been fascinated by the night sky, from ancient civilizations that worshiped celestial bodies to modern astronomers using advanced telescopes and spacecraft. Among the myriad mysteries of space, the gas giants Jupiter and Saturn stand out due to their unique and persistent atmospheric patterns. These geometric configurations have led to intriguing hypotheses about their origins, including the possibility of alien communication.
Jupiter and Saturn, the two largest planets in our solar system, have fascinated scientists for centuries. Their massive sizes, diverse moons, and intricate ring systems make them subjects of extensive study. However, one of the most captivating features of these gas giants is their atmospheric phenomena, particularly the geometric patterns observed at their poles. The geometric patterns on Jupiter and Saturn, revealed through missions like Juno and Cassini, offer a tantalizing hint at potential extraterrestrial intelligence. This document explores these patterns, their scientific explanations, and the bold proposal to construct a geometric signal on Earth's South Pole to reciprocate potential alien communication.
Historical Context
The exploration of the outer planets began in earnest with the Pioneer and Voyager missions in the 1970s and 1980s. These early missions provided the first close-up images of Jupiter and Saturn, revealing their complex atmospheres and extensive magnetospheres. The data collected by these missions laid the groundwork for future exploration and set the stage for more sophisticated missions like Galileo, Juno, and Cassini.
Jupiter, the largest planet in our solar system, has been studied extensively due to its massive size and strong magnetic field. Its Great Red Spot, a gigantic storm larger than Earth, has been observed for over 350 years. Similarly, Saturn's rings have intrigued scientists since Galileo first observed them in 1610. However, it is the lesser-known polar regions of these planets that have recently captured the attention of researchers due to their unique geometric patterns.
Scientific Exploration
The study of Jupiter and Saturn has been greatly enhanced by the advancements in space exploration technology. The Galileo mission, which orbited Jupiter from 1995 to 2003, provided detailed data on the planet's atmosphere, moons, and magnetosphere. The Cassini mission, which orbited Saturn from 2004 to 2017, delivered unprecedented insights into the planet's rings, moons, and atmospheric phenomena. These missions laid the groundwork for the current Juno mission, which has been orbiting Jupiter since 2016, and continues to provide valuable data on the planet's atmospheric dynamics and magnetic field.
The Juno mission, launched by NASA in 2011, has been particularly instrumental in uncovering the mysteries of Jupiter's polar regions. Equipped with a suite of scientific instruments, Juno has provided high-resolution images and detailed measurements of Jupiter's atmosphere, magnetic field, and gravitational field. These data have revealed the presence of stable, geometric patterns in the planet's polar cyclones, challenging our understanding of atmospheric dynamics on gas giants.
Similarly, the Cassini mission to Saturn, which ended in 2017, provided a wealth of information about the planet's atmosphere and its iconic hexagonal storm at the north pole. Cassini's high-resolution images and infrared measurements have allowed scientists to study the hexagon in unprecedented detail, revealing its structure, dynamics, and possible formation mechanisms.
The Mystery of Geometric Patterns
One of the most intriguing discoveries of the Juno and Cassini missions is the presence of stable geometric patterns in the atmospheres of Jupiter and Saturn. These patterns, which include polygons with a varying number of sides, have been observed in the cyclonic storms at the poles of these planets. On Jupiter, the north pole features a central cyclone surrounded by eight smaller cyclones arranged in an octagonal pattern, while the south pole has a central cyclone surrounded by five smaller cyclones arranged in a pentagonal pattern.
On Saturn, the north pole is dominated by a massive hexagonal storm, which has puzzled scientists since its discovery by the Voyager missions.
The stability and regularity of these patterns are remarkable, suggesting that they are maintained by complex interactions between atmospheric dynamics and the planets' magnetic fields. Understanding the formation and stability of these geometric patterns is a major challenge for planetary scientists, and it has led to a variety of hypotheses, ranging from purely natural explanations to the possibility of intentional design by an advanced extraterrestrial civilization.
The Hypothesis of Extraterrestrial Communication
The hypothesis that these geometric patterns could be intentional signals from an advanced extraterrestrial civilization is both intriguing and controversial. Proponents of this hypothesis argue that the patterns' regularity and stability suggest a level of control that is unlikely to occur naturally. They propose that an advanced civilization might use these patterns to communicate with other intelligent beings, using the planets' atmospheres as a canvas for their messages.
This idea, while speculative, is not without precedent. Throughout history, humans have used geometric patterns as a means of communication, from ancient crop circles to modern radio signals. The use of geometry as a universal language is based on the idea that mathematical principles are likely to be understood by any advanced civilization, regardless of their biological or cultural differences.
The hypothesis of extraterrestrial communication is further supported by the fact that these patterns are located at the poles of the planets, where they would be most visible to observers from space. This strategic placement suggests that the patterns might be intended to attract attention and signal the presence of intelligent life.
The Proposal to Build a Geometric Signal on Earth: Orbital Number
In light of these discoveries, a bold proposal has been put forward to construct a geometric signal on Earth's South Pole, using the same principles observed in the patterns on Jupiter and Saturn. The signal seems to be that the planet’s orbital number corresponds to the number of sides represented on the polygon displayed on a planet’s pole(s). 6th planet: 6-sided hexagon; 5th/8 planets: pentagon & octagon.
The idea is to create a large, stable geometric pattern that would be visible from space, serving as a signal to any potential extraterrestrial observers.
This proposal involves significant engineering challenges, including the harsh conditions of the Antarctic environment and the need for precise geometric construction. However, recent advancements in technology, such as autonomous drones and advanced materials, make it feasible to create such a structure. The proposed signal would consist of a large triangle at the South Pole that could be easily recognized from space.
Significance and Implications
The construction of a geometric signal on Earth would have profound implications for our understanding of extraterrestrial communication and our place in the universe. If successful, it would demonstrate our ability to create stable, large-scale geometric patterns and signal our presence to potential extraterrestrial observers. It would also serve as a testament to human ingenuity and our desire to explore and understand the cosmos.
Furthermore, the project would provide valuable data on the formation and stability of geometric patterns in planetary atmospheres, contributing to our understanding of atmospheric dynamics on gas giants. It would also serve as a catalyst for further research and exploration, inspiring future missions to study the polar regions of Jupiter and Saturn in greater detail.
Challenges and Future Directions
The proposal to build a geometric signal on Earth's South Pole is ambitious and faces several challenges. The harsh conditions of the Antarctic environment, including extreme cold, high winds, and isolation, pose significant logistical and engineering challenges. Additionally, the need for precise geometric construction requires advanced materials and technology.
Despite these challenges, the project has the potential to push the boundaries of human achievement and inspire future generations of scientists and engineers. It represents a bold step in the quest for extraterrestrial communication and our desire to explore and understand the universe.
Future research will focus on refining the design of the geometric signal, developing the necessary technology for construction, and studying the potential impact of the project on the Antarctic environment. Additionally, continued observations of Jupiter and Saturn will provide valuable data on the formation and stability of geometric patterns in planetary atmospheres, contributing to our understanding of these enigmatic features.
The discovery of stable geometric patterns in the atmospheres of Jupiter and Saturn has opened up new avenues of research and speculation about the possibility of extraterrestrial communication. The hypothesis that these patterns could be intentional signals from an advanced civilization is both intriguing and controversial, challenging our understanding of planetary science and our place in the universe.
The idea of responding to this cosmic “Primer” represents a bold step in the quest for extraterrestrial communication, pushing the boundaries of human achievement and technological capability. While the project faces significant challenges, it has the potential to provide valuable data on atmospheric dynamics, inspire future research, and signal our presence to potential extraterrestrial observers.
As we continue to explore the mysteries of the cosmos, the study of geometric patterns on Jupiter and Saturn will remain a fascinating and important area of research, offering new insights into the dynamics of planetary atmospheres and the potential for communication with intelligent life beyond Earth.
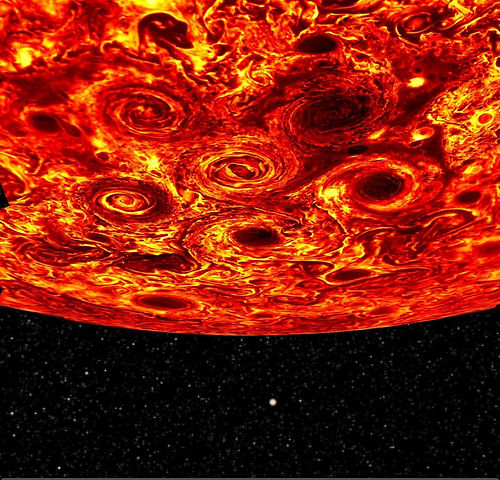
Section 2: Geometric Patterns on Jupiter and Saturn
Cyclones at Jupiter's Poles
Recent Discoveries
NASA's Juno mission has significantly advanced our understanding of Jupiter's atmospheric dynamics. Launched in 2011, Juno entered Jupiter's orbit in 2016 and has since provided detailed observations of the planet's poles. These observations have revealed the presence of stable geometric patterns in the form of cyclones. At Jupiter's north pole, there is a central cyclone surrounded by eight others arranged in an octagonal pattern. Similarly, the south pole features a central cyclone surrounded by five smaller cyclones arranged in a pentagonal pattern.
These cyclones are massive, each spanning several thousand kilometers in diameter. The stability and regularity of these patterns challenge our understanding of atmospheric dynamics on gas giants. The patterns have persisted for years, indicating a complex and stable atmospheric system.
Formation and Stability
The formation and stability of these cyclones are subjects of ongoing research. Current models suggest that anticyclonic rings, or counter-rotating wind patterns, play a crucial role in maintaining these geometric structures. These rings provide a balancing force that prevents the cyclones from merging into a single chaotic system.
Advanced computational models simulate the atmospheric conditions on Jupiter, considering factors such as the planet's rapid rotation, differential heat distribution, and deep atmospheric currents. These simulations indicate that the interplay of these forces can create stable geometric patterns that persist over time. The dynamics involve complex fluid motion driven by Jupiter's internal heat and rapid rotation. Researchers have proposed that the cyclones are stabilized by the interaction between deep convective cells and the planet's magnetic field.
Implications for Planetary Science
The discovery of stable geometric patterns on Jupiter challenges our understanding of planetary atmospheres. It suggests that such patterns might be more common in the universe than previously thought, potentially indicating similar processes at work on other gas giants or exoplanets. This insight opens new avenues for comparative planetology, where scientists can study atmospheric dynamics across different planetary environments.
Scientific Exploration and Ongoing Research
Detailed studies have revealed that these cyclones are not just superficial phenomena but extend deep into Jupiter's atmosphere. The depth of these cyclones influences their stability and persistence. Furthermore, the interaction between these cyclones and Jupiter’s magnetic field is another area of active research. Scientists hypothesize that the magnetic field could play a role in stabilizing these structures.
Juno's ongoing mission continues to collect data, providing new insights into the thermal dynamics and chemical composition of Jupiter's atmosphere. This data is crucial for refining models and understanding the energy sources driving these cyclonic structures. Researchers are particularly interested in the role of ammonia and water vapor in the formation and maintenance of these cyclones.
Saturn's Hexagonal Storm
Historical Background
Saturn's hexagon was first discovered by the Voyager spacecraft in the early 1980s and later studied extensively by the Cassini mission. This hexagonal storm, located at the planet's north pole, has puzzled scientists for decades due to its regular shape and persistence.
The hexagon spans approximately 30,000 kilometers across and is a unique feature not seen on any other planet in the solar system. The mystery of its formation and stability has led to numerous hypotheses and extensive scientific inquiry.
Mechanisms of Formation
The hexagon is believed to be a standing wave pattern, a type of atmospheric phenomenon where wave structures remain stationary relative to the rotating planet. This pattern is thought to result from the differential rotation of Saturn's atmosphere, where different latitudes rotate at different speeds. Fluid dynamics models suggest that the interaction between deep atmospheric jets and shallower layers can create the necessary conditions for such a stable geometric feature.
High-resolution observations from Cassini have revealed intricate details of the hexagon's structure, including its vertical extent and the presence of smaller vortices within its boundary. These observations have been critical in developing models to explain how the hexagon can remain stable over decades.
Observational Data and Analysis
Cassini's extensive data collection has provided detailed information about the hexagon's structure and dynamics. High-resolution images and thermal infrared measurements have revealed that the hexagon extends deep into Saturn's atmosphere and is associated with a strong jet stream. These observations have been used to refine models of the hexagon's formation, incorporating the effects of atmospheric turbulence and wave propagation.
Thermal imaging has shown that the hexagon's boundaries are warmer than its center, indicating a complex interaction of thermal dynamics and atmospheric waves. This temperature gradient is essential for maintaining the stability of the hexagonal shape, as it drives the jet stream that defines the hexagon's boundaries.
Continued Study and Technological Advances
Recent technological advancements have allowed scientists to study Saturn’s hexagon in greater detail. High-resolution spectroscopy and advanced atmospheric modeling techniques are providing new insights into the chemical composition and thermal dynamics of the hexagon. These studies suggest that seasonal changes on Saturn might also affect the stability and appearance of the hexagon, adding another layer of complexity to our understanding.
The ongoing analysis of data from Cassini's mission continues to shed light on the interplay between Saturn's atmospheric dynamics and the hexagonal pattern. Researchers are particularly interested in the potential role of Saturn's magnetic field and internal heat sources in maintaining this structure.
Theoretical Models and Stability Mechanisms
Introduction to Atmospheric Dynamics
The study of atmospheric dynamics on gas giants like Jupiter and Saturn involves understanding the complex interactions between thermal convection, rotational forces, and magnetic fields. These interactions give rise to the unique geometric patterns observed at the poles of these planets.
Rotational Dynamics and Vortex Stability
One of the key factors contributing to the stability of these geometric patterns is the rapid rotation of Jupiter and Saturn. The Coriolis effect, resulting from this rotation, plays a crucial role in shaping the atmospheric circulation and stabilizing large-scale vortices.
Researchers have developed models to simulate the behavior of these vortices under various conditions. These models consider the effects of differential rotation, where different latitudes on the planet rotate at different speeds, leading to the formation of distinct jet streams and vortices.
Thermal Convection and Heat Transfer
Thermal convection within the atmospheres of Jupiter and Saturn is driven by the internal heat generated by these planets. This convection creates upwellings of warmer, less dense gas, which rise and interact with the cooler, denser gas above. These interactions generate turbulence and contribute to the formation and stability of cyclonic structures.
Magnetic Field Interactions
The magnetic fields of Jupiter and Saturn are among the strongest in the solar system and play a significant role in their atmospheric dynamics. The interaction between the magnetic field and atmospheric currents can influence the formation and stability of geometric patterns. Studies suggest that the magnetic field can induce electromagnetic forces that help stabilize the cyclonic structures observed at the poles.
Comparative Analysis of Jupiter and Saturn
Similarities in Atmospheric Dynamics
Both Jupiter and Saturn exhibit complex atmospheric dynamics characterized by rapid rotation, strong magnetic fields, and significant internal heat. These similarities contribute to the formation of stable geometric patterns in their polar regions. Understanding the common mechanisms behind these patterns can provide insights into the general principles of atmospheric dynamics on gas giants.
Differences in Geometric Patterns
Despite their similarities, the geometric patterns observed on Jupiter and Saturn differ in several ways. Jupiter's cyclones are arranged in octagonal and pentagonal patterns at the north and south poles, respectively, while Saturn's north pole features a single, massive hexagonal storm. These differences suggest that additional factors, such as the specific properties of each planet's atmosphere and magnetic field, play a role in shaping these patterns.
Implications for Exoplanetary Research
The discovery of stable geometric patterns on Jupiter and Saturn has significant implications for the study of exoplanets. As researchers continue to discover and characterize gas giants in other star systems, the principles learned from studying our own solar system's giants can be applied to understand the atmospheric dynamics of these distant worlds. The presence of similar geometric patterns on exoplanets could indicate common processes at work and provide clues about their atmospheric composition and behavior.
Future Directions in Research
The study of geometric patterns on Jupiter and Saturn is an ongoing field of research with many unanswered questions. Future missions and observations will aim to gather more data on these patterns and their underlying mechanisms. Advances in computational modeling and observational technology will enable scientists to test new hypotheses and refine existing models, deepening our understanding of the atmospheric dynamics on gas giants.
Researchers are also exploring the potential for collaborative international missions to study the outer planets in greater detail. Such missions could include orbiters, atmospheric probes, and even landers designed to explore the moons of Jupiter and Saturn, which are themselves intriguing worlds with complex interactions with their parent planets' magnetic fields and atmospheres.
The geometric patterns observed at the poles of Jupiter and Saturn represent one of the most intriguing mysteries in planetary science. The stable cyclones and hexagonal storm challenge our understanding of atmospheric dynamics and suggest complex interactions between thermal convection, rotational forces, and magnetic fields. These patterns not only provide insights into the behavior of gas giants but also raise intriguing possibilities about the potential for extraterrestrial communication.
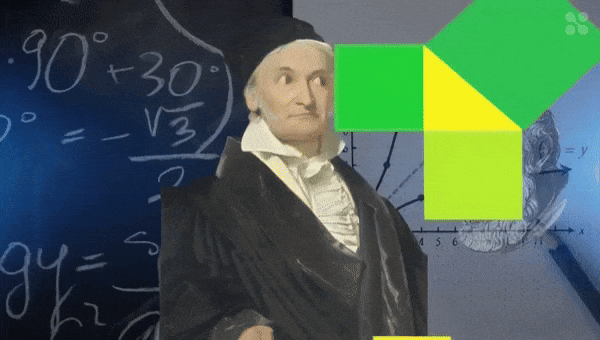
Section 3: Historical Perspectives on Alien Communication
Gauss's Triangle Proposal
Mathematical Foundations
The renowned mathematician Carl Friedrich Gauss is often credited with one of the earliest proposals for signaling extraterrestrial intelligences through geometric means. In the early 19th century, Gauss suggested that humanity could communicate its presence to potential alien civilizations by creating large geometric figures on Earth. He specifically proposed the construction of a giant right-angled triangle in Siberia, using fields of different kinds of grain to create the edges. This triangle would be visible from space, thereby signaling intelligent life on Earth.
Gauss's idea was rooted in the belief that geometric shapes, based on universal mathematical principles, would be understood by any intelligent beings capable of observing them. The simplicity and universality of geometric forms like triangles, squares, and circles make them ideal candidates for such communication attempts. Gauss argued that any intelligent civilization with knowledge of mathematics would recognize these shapes as artificial constructs, indicating the presence of intelligent life.
The concept of using large-scale geometric figures as a means of extraterrestrial communication has a certain timeless appeal. It relies on the assumption that mathematics is a universal language, transcending the specificities of culture and biology. This assumption is foundational to many modern SETI (Search for Extraterrestrial Intelligence) initiatives, which often employ mathematical and geometric principles in their search strategies.
Modern Interpretations
In contemporary times, Gauss's proposal is frequently revisited and reinterpreted within the broader context of SETI. Modern interpretations of Gauss's idea involve using advanced technology to create even more intricate and detectable signals. For instance, large arrays of lights or mirrors could be arranged to form geometric patterns that would be visible from space. These patterns could then be detected by extraterrestrial observers using telescopes or other sensing equipment.
One such modern reinterpretation is the concept of a "geoglyph," which involves creating large-scale designs or motifs on the Earth's surface. These geoglyphs could be made using a variety of materials, from rocks and soil to reflective metals or lights. The goal would be to create patterns that are unmistakably artificial and geometrically precise, making them easily recognizable as signals from an intelligent civilization.
The idea of using geometry as a means of communication is also explored in the field of radio SETI. Researchers have proposed encoding messages in geometric patterns of radio signals, which could be more easily distinguished from natural cosmic noise. For example, a sequence of pulses representing prime numbers or the digits of π (pi) could be transmitted, as these sequences are unlikely to occur naturally and would signify an intelligent origin.
Evolution of SETI and Mathematical Signals
Early Efforts
The search for extraterrestrial intelligence (SETI) has evolved significantly since Gauss's time. Early efforts were primarily theoretical, with scientists speculating on the best ways to communicate with alien civilizations. These early speculations often involved the use of visible light or large-scale constructions, such as the proposed triangle in Siberia.
In the mid-20th century, the advent of radio astronomy provided a new avenue for SETI. Scientists like Frank Drake and Carl Sagan pioneered the use of radio telescopes to listen for signals from other civilizations. The famous Drake Equation, formulated by Frank Drake in 1961, provided a framework for estimating the number of active, communicative extraterrestrial civilizations in the Milky Way galaxy. This equation considers factors such as the rate of star formation, the fraction of stars with planets, and the likelihood of life developing on those planets.
Modern SETI Initiatives
Today, SETI initiatives employ a variety of advanced technologies to search for extraterrestrial signals. Radio telescopes remain a primary tool, with projects like the Breakthrough Listen initiative scanning the skies for potential signals. Breakthrough Listen, launched in 2015, is the most comprehensive SETI program to date, using some of the world's largest radio telescopes to monitor the 1,000,000 nearest stars to Earth and the center of our galaxy.
In addition to radio SETI, optical SETI has emerged as a complementary approach. Optical SETI involves searching for brief flashes of light, known as laser pulses, which could be sent by advanced civilizations using powerful lasers. Projects like the Laser SETI program aim to detect these pulses by monitoring large areas of the sky with high sensitivity.
Mathematical signals remain a cornerstone of modern SETI strategies. Researchers continue to explore ways to encode messages in mathematical patterns, such as prime numbers, Fibonacci sequences, or geometric shapes, that would stand out against the background noise of the cosmos. The assumption is that any civilization capable of interstellar communication would understand these patterns, making them a universal language for SETI.
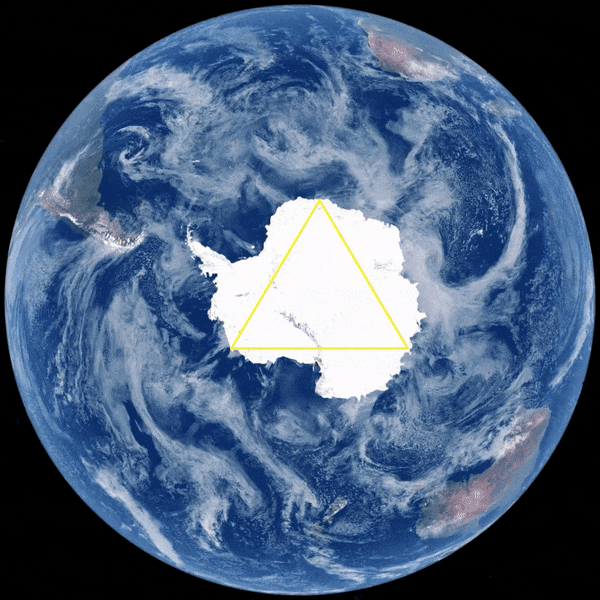
Section 4: The Primer Concept
Deciphering the Patterns
The geometric shapes observed on Jupiter and Saturn have led to the hypothesis that these patterns might be intentional signals from an advanced alien civilization. This idea, known as "The Primer," posits that the aliens use polygons with a number of sides corresponding to the planet's position in the solar system to indicate their presence.
The notion of using planetary features as signals is intriguing and builds on the idea that intelligent civilizations might use natural objects in space to communicate. The regularity and stability of these patterns, such as the hexagon on Saturn and the polygons on Jupiter, suggest a level of control that could indicate artificial manipulation. Scientists are examining the possibility that these features could be part of a complex message or code intended for other intelligent beings to decipher.
Deciphering these patterns involves analyzing their geometric properties, such as symmetry, angles, and proportions. Researchers are also exploring the possibility that the patterns could encode information in their arrangement or changes over time. For example, the presence of specific shapes at different locations or their evolution might convey a message or instructions for further exploration.
Implications for Alien Communication
If The Primer hypothesis is correct, it implies a sophisticated understanding of geometry and planetary science by the signaling civilization. It also suggests a peaceful intent, as the patterns serve as an invitation to recognize and understand rather than an aggressive act.
The implications for alien communication are profound. Firstly, it would indicate that advanced civilizations use a common language of mathematics and geometry, reinforcing the idea that these principles are universal. Secondly, it would suggest that intelligent beings are actively seeking to make contact with other civilizations, using natural phenomena as a medium for their messages. Finally, it would provide a framework for understanding and interpreting potential signals from other planets or moons in our solar system and beyond.
The historical perspectives on alien communication, from Gauss's geometric proposals to modern SETI initiatives, demonstrate the enduring appeal of using mathematics and geometry as a universal language. The discovery of geometric patterns on Jupiter and Saturn adds a new dimension to this quest, suggesting that such patterns could be intentional signals from an advanced civilization. As research continues, these historical and modern approaches will guide our efforts to understand and communicate with potential extraterrestrial intelligences, paving the way for a new era of exploration and discovery.
Hypotheses on Extraterrestrial Communication
The hypothesis that these geometric patterns could be intentional signals from an advanced civilization is based on several key observations:
1. Regularity and Stability: The geometric patterns on Jupiter and Saturn are highly regular and stable, persisting over long periods. This suggests a level of control and precision that may be beyond natural atmospheric processes.
2. Mathematical Universality: Mathematics is often considered a universal language. The use of geometric shapes, which are based on fundamental mathematical principles, could be a way for an advanced civilization to communicate across vast distances. These shapes would be recognizable to any intelligent beings with an understanding of geometry.
3. Strategic Placement: The patterns are located at the poles of Jupiter and Saturn, making them visible to observers from space. This strategic placement suggests that they might be intended to attract attention and signal the presence of intelligent life.
Framework for Understanding
The Primer concept provides a framework for interpreting potential signals from other planets and moons in our solar system and beyond. By examining the geometric properties of these patterns, scientists can develop hypotheses about their origin and meaning. This involves analyzing the shapes, symmetry, and arrangement of the patterns, as well as any changes over time that might convey additional information.
Technological and Scientific Implications
The discovery of geometric patterns on Jupiter and Saturn has significant implications for our understanding of extraterrestrial communication and planetary science. It challenges our current models of atmospheric dynamics and suggests that advanced civilizations might use natural phenomena as a medium for their messages.
In addition, the study of these patterns could lead to new technological advancements. For example, the development of more sophisticated observational instruments and data analysis techniques could improve our ability to detect and interpret signals from other planets. It could also inspire new approaches to communication and exploration, both within our solar system and beyond.
Future Research Directions
Continued Observations
Future research will involve continued observations of Jupiter and Saturn to gather more data on the geometric patterns at their poles. This will require the use of advanced telescopes and space missions equipped with high-resolution imaging and spectroscopic instruments. These observations will help scientists refine their models of pattern formation and stability, providing a better understanding of the underlying mechanisms.
Comparative Planetology
Comparative planetology, the study of similarities and differences between planets, will play a crucial role in future research. By comparing the geometric patterns on Jupiter and Saturn with those on other gas giants and exoplanets, scientists can identify common processes and unique features. This comparative approach will provide insights into the general principles of atmospheric dynamics and the potential for extraterrestrial communication.
Interdisciplinary Collaboration
The study of The Primer concept will benefit from interdisciplinary collaboration between planetary scientists, astrophysicists, mathematicians, and engineers. By combining expertise from different fields, researchers can develop more comprehensive models and innovative approaches to studying and interpreting geometric patterns on other planets.
The Role of Space Missions
Current and Upcoming Missions
Several current and upcoming space missions will contribute to the study of geometric patterns on Jupiter, Saturn, and other gas giants. NASA's Juno mission, which has been orbiting Jupiter since 2016, continues to provide valuable data on the planet's polar regions. The European Space Agency's (ESA) Jupiter Icy Moons Explorer (JUICE) mission, launched in 2023, will study Jupiter's atmosphere and its largest moons.
For Saturn, future missions could include orbiters and atmospheric probes designed to study the planet's hexagonal storm in greater detail. These missions will provide high-resolution data on the structure, dynamics, and composition of Saturn's atmosphere, helping scientists understand the mechanisms behind the hexagon and its potential as a communication signal.
The Search for Exoplanetary Patterns
Exoplanetary Research
The study of geometric patterns on gas giants extends beyond our solar system. With the discovery of thousands of exoplanets, researchers are now looking for similar patterns on distant worlds. The presence of geometric patterns on exoplanets could indicate common atmospheric processes or even the presence of advanced civilizations using similar communication methods.
Advanced Observational Techniques
Advanced observational techniques, such as high-resolution spectroscopy and direct imaging, will play a crucial role in the search for geometric patterns on exoplanets. Instruments like the James Webb Space Telescope (JWST) and the Extremely Large Telescope (ELT) will provide unprecedented capabilities for studying the atmospheres of distant planets. By detecting and analyzing potential geometric patterns, scientists can gain insights into the atmospheric dynamics and possible signs of extraterrestrial life on these worlds.
The Primer concept represents a fascinating and provocative hypothesis in the search for extraterrestrial intelligence. By examining the geometric patterns on Jupiter and Saturn, researchers are exploring the possibility that these features could be intentional signals from an advanced civilization. The implications of this hypothesis are profound, challenging our understanding of planetary science and opening new avenues for research and exploration.
As technology and observational capabilities continue to advance, future missions and studies will provide more data and insights into these enigmatic patterns. By combining the efforts of planetary scientists, astrophysicists, and mathematicians, we can further unravel the mysteries of The Primer and its potential as a universal language for communicating with intelligent beings across the cosmos.
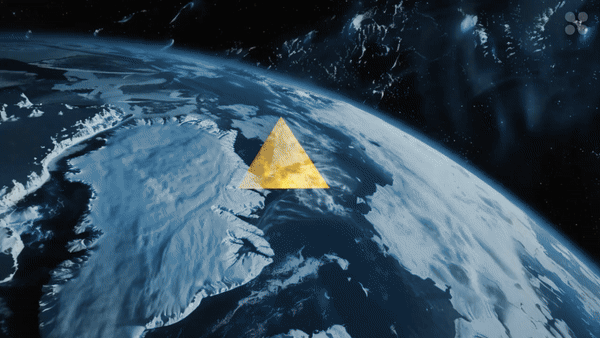
Section 5: Building the Primer Beacon on Earth
Design and Engineering
Conceptual Design
The proposal to build a geometric signal on Earth’s South Pole involves the creation of a massive triangular structure. This "Primer Beacon" aims to signal to extraterrestrial intelligences that humans have recognized and understood their geometric messages. The beacon would consist of three sides, each potentially several thousand kilometers long, forming a precise equilateral triangle. The construction would use materials capable of withstanding the extreme Antarctic environment, such as carbon fiber composites and advanced polymers. The triangle would be designed to be highly visible from space, possibly incorporating reflective materials or lighting systems to ensure its detectability.
Engineering Challenges
Building a large structure in the harsh conditions of Antarctica presents numerous engineering challenges. The extreme cold, high winds, and remote location require innovative solutions for both the construction process and the long-term maintenance of the beacon.
-
Material Selection: Materials must be chosen for their durability and resistance to extreme cold. Carbon fiber composites, known for their strength and lightweight properties, are ideal. Additionally, materials with low thermal expansion coefficients are necessary to prevent structural deformation.
-
Foundation and Stability: Establishing a stable foundation on the icy terrain is crucial. This involves drilling into the ice to anchor the structure securely. Advanced geotechnical engineering techniques, such as deep foundations and ground freezing methods will be employed to ensure stability.
-
Construction Techniques: The use of autonomous drones and robots will be essential for construction. These robots, equipped with AI-guided systems, can operate continuously in the harsh environment, ensuring precise placement of materials. Modular construction techniques will allow for components to be prefabricated and assembled on-site, reducing the need for extensive human presence.
-
Energy Requirements: The beacon will require a reliable energy source for construction and operation. Renewable energy solutions, such as solar panels and wind turbines, will be essential. Additionally, advanced energy storage systems such as BetaVolt - which uses Ni-63 to power a nuclear battery which lasts for decades - will ensure continuous power supply during the polar night.
Technological Innovations
Several technological innovations will be employed to overcome the challenges of constructing the Primer Beacon:
-
Autonomous Robotics: Robots equipped with AI and advanced sensors will handle construction tasks. These robots can work in extreme conditions, reducing the risks to human workers and increasing efficiency.
-
Advanced Materials: The use of cutting-edge materials like aerogels for insulation and carbon nanotubes for structural elements will enhance the durability and stability of the beacon.
-
Energy Harvesting: Innovative energy harvesting techniques, such as piezoelectric systems that convert mechanical energy from wind and ice movement into electricity, will complement traditional renewable energy sources.
-
Remote Monitoring: The beacon will be equipped with sensors to monitor structural integrity and environmental conditions. Data will be transmitted to control centers via satellite, allowing for real-time monitoring and maintenance planning.
Technological Requirements
Satellite Imaging and Geometric Precision
Ensuring the geometric precision of the Primer Beacon is crucial for its visibility and effectiveness as a signal. Satellite imaging will play a key role in both the construction and monitoring phases. High-resolution satellite images will be used to plan the layout, verify alignment, and detect any deformations over time.
-
Geospatial Analysis: Advanced geospatial analysis techniques will be employed to map the terrain and plan the construction. This includes using LIDAR (Light Detection and Ranging) to create detailed topographic maps and identify suitable locations for the beacon.
-
Real-Time Monitoring: During construction, real-time satellite imagery will be used to monitor progress and ensure accuracy. Any deviations from the planned design can be quickly identified and corrected.
-
Long-Term Surveillance: After completion, the beacon will be continuously monitored using satellite imagery. This will help detect any structural issues or environmental impacts, allowing for timely maintenance and adjustments.
Renewable Energy Solutions
The harsh Antarctic environment necessitates reliable and sustainable energy solutions to power the beacon. Renewable energy sources, such as solar and wind power, will be the primary energy providers.
-
Solar Power: Solar panels will be strategically placed to maximize exposure to sunlight during the Antarctic summer. Advances in solar technology, such as high-efficiency photovoltaic cells and bifacial panels that capture sunlight from both sides will enhance energy production.
-
Wind Power: Wind turbines will harness the strong and consistent winds of Antarctica. Vertical-axis wind turbines, which are more effective in turbulent wind conditions, will be preferred. These turbines can operate efficiently even in the extreme cold.
-
Energy Storage: Advanced energy storage solutions, such as lithium-ion and solid-state batteries, will store excess energy produced during periods of high solar and wind activity. This stored energy will ensure continuous power supply during the polar night and periods of low energy generation.
-
Hybrid Systems: A hybrid energy system combining solar, wind, and other renewable sources will provide a reliable and resilient energy supply. This system will include smart grid technology to optimize energy distribution and minimize waste.
Potential Benefits and Impact
Scientific Advancements
The construction of the Primer Beacon will have significant scientific benefits. It will provide a unique opportunity to study the interactions between advanced materials, energy systems, and extreme environmental conditions. The data collected will contribute to our understanding of material science, renewable energy, and autonomous robotics in harsh environments.
-
Material Science: Testing and monitoring advanced materials in the Antarctic environment will yield valuable insights into their performance and durability. This knowledge can be applied to future construction projects in extreme conditions, both on Earth and in space.
-
Renewable Energy Research: The integration of renewable energy systems in the beacon will serve as a real-world testbed for innovative energy solutions. The findings will contribute to the development of more efficient and resilient renewable energy technologies.
-
Robotic Engineering: The use of autonomous robots for construction and maintenance will provide critical data on their capabilities and limitations. This information will drive advancements in robotics, particularly for applications in remote and hazardous locations.
Technological Innovation
The Primer Beacon project will push the boundaries of current technology, driving innovation in several fields. The challenges of constructing and maintaining the beacon in Antarctica will lead to the development of new technologies and techniques that can be applied to other areas.
-
Autonomous Systems: The development of autonomous construction and maintenance systems will have wide-ranging applications. These systems can be used in other remote and hazardous environments, such as deep-sea exploration, disaster response, and space missions.
-
Advanced Construction Techniques: The Primer Beacon project will pioneer new construction techniques, such as modular and prefabricated structures, that can be deployed quickly and efficiently in challenging conditions. These techniques can be applied to urban development, disaster recovery, and space colonization.
-
Environmental Monitoring: The sensors and monitoring systems used in the beacon will provide valuable data on environmental conditions in Antarctica. This information will contribute to our understanding of climate change and its impacts on polar regions.
Global Collaboration
The Primer Beacon project will foster international collaboration and cooperation. The ambitious nature of the project will require expertise and resources from multiple countries, promoting a spirit of global unity in the pursuit of scientific and technological advancement.
-
Collaborative Research: Scientists and engineers from around the world will work together on the project, sharing knowledge and expertise. This collaboration will strengthen international partnerships and promote the exchange of ideas.
-
Educational Opportunities: The project will provide educational opportunities for students and researchers, inspiring the next generation of scientists and engineers. Programs and internships associated with the project will offer hands-on experience and training in advanced technologies.
-
Public Engagement: The Primer Beacon will capture the public's imagination, raising awareness of the potential for extraterrestrial communication and the importance of scientific exploration. Outreach programs and public events will engage people of all ages in the excitement of the project.
Environmental and Ethical Considerations
Environmental Impact
Constructing the Primer Beacon in Antarctica requires careful consideration of the environmental impact. The Antarctic region is a pristine and fragile ecosystem, and any human activity must be conducted with the utmost care to minimize harm.
-
Environmental Assessment: A comprehensive environmental assessment will be conducted to evaluate the potential impacts of the project. This assessment will include studies on the effects of construction, energy generation, and long-term operation on the local environment.
-
Sustainable Practices: The project will implement sustainable practices to minimize its environmental footprint. This includes using renewable energy sources, reducing waste, and employing eco-friendly materials and construction methods.
-
Monitoring and Mitigation: Continuous environmental monitoring will be conducted to detect and mitigate any adverse impacts. This monitoring will include assessments of air and water quality, wildlife populations, and ice stability.
Ethical Considerations
The ethical implications of constructing the Primer Beacon must also be carefully considered. This includes the potential impacts on indigenous communities, the use of advanced technologies, and the broader implications of signaling extraterrestrial intelligences.
-
Indigenous Rights: Although Antarctica is not home to indigenous human populations, it is governed by the Antarctic Treaty System, which emphasizes the protection of the environment and the promotion of peaceful scientific exploration. The project must adhere to the principles of the treaty and ensure that its activities are conducted responsibly.
-
Technology Ethics: The use of autonomous robots and advanced materials raises ethical questions about the potential risks and benefits of these technologies. The project will adhere to ethical guidelines for the development and deployment of technology, ensuring that it is used responsibly and for the benefit of humanity.
-
Extraterrestrial Communication: The decision to signal extraterrestrial intelligences carries significant ethical implications. This includes considerations of the potential risks and benefits of making contact with an advanced civilization. The project will involve consultations with experts in ethics, astronomy, and international policy to ensure that the decision is made thoughtfully and responsibly.
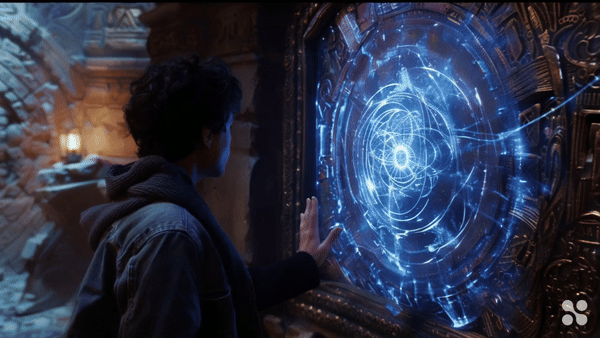
Section 6: Antarctic Colonization and $PRIM Currency
Incentives and Economics
The Concept of $PRIM Currency
The proposal to build the Primer Beacon on Earth’s South Pole involves significant logistical and financial challenges. To address these, the concept of $PRIM, a new digital currency, has been introduced. $PRIM aims to incentivize participation in the project and facilitate economic activities in Antarctica. By creating a robust economic system, $PRIM seeks to attract individuals and organizations to contribute to the construction and maintenance of the Primer Beacon.
Economic Model
The economic model for $PRIM is based on several key principles:
1. Decentralization: $PRIM will be a decentralized cryptocurrency, leveraging the Solana blockchain’s technology to ensure transparency, security, and trust. Decentralization will also enable peer-to-peer transactions, reducing the need for intermediaries and lowering transaction costs.
2. Incentives for Participation: Individuals and organizations that contribute to the Primer Beacon project will be rewarded with $PRIM tokens. This includes scientists, engineers, construction workers, and support staff. The tokens can be used to purchase goods and services within the Antarctic colony or traded on global cryptocurrency exchanges.
3. Sustainable Economy: The $PRIM economy will be designed to promote sustainability and environmental stewardship. Economic activities will be regulated to minimize their impact on the Antarctic environment, and a portion of $PRIM tokens will be allocated to fund conservation and research initiatives.
4. Integration with Global Markets: $PRIM will be integrated with global cryptocurrency markets, allowing for easy conversion to other currencies. This will facilitate trade and investment, making it attractive for businesses to operate in Antarctica.
Initial Coin Offering (ICO)
To raise funds for the Primer Beacon project, an Initial Coin Offering (ICO) will be conducted. The ICO will offer $PRIM tokens to investors in exchange for traditional currencies or other cryptocurrencies. The funds raised will be used to finance the construction, maintenance, and operation of the Primer Beacon.
1. Marketing and Outreach: A comprehensive marketing campaign will be launched to raise awareness about the ICO and the Primer Beacon project. This will include social media, online advertisements, and partnerships with influential figures in the cryptocurrency and scientific communities.
2. Investor Protections: To attract investors, robust protections will be put in place. These include transparent reporting of project progress, regular audits of funds, and a clear roadmap outlining milestones and timelines.
Tokenomics
-
1,000,000,000 $PRIM
-
25% to Liquidity Pool
-
25% to Research
-
25% to Infrastructure
-
10% to Marketing
-
5% to Presale
-
5% to DogWifHat Founder Patrick Bateman
-
5% to Founder Paul Hallelujah
-
Mint Authority Revoked
-
CA: 6rbCpRkJGmJgNLNBkmUe5oPzCEJy1JGG34fyUPPHf6DW
Presale
-
1 Sol minimum
-
20 Sol maximum
-
Soft Cap 100 Sol
-
Hard Cap 1000 Sol
-
Send to: Et9ji13tWfnAcgD7zJDm6RCaw6KnSxrXWN8jVdSYyTgw
Challenges and Solutions
Logistical Challenges
Building and maintaining a colony in Antarctica involves numerous logistical challenges, including transportation, supply chain management, and the harsh environmental conditions.
1. Transportation: The transportation of materials, equipment, and personnel to Antarctica is a major challenge. Solutions include the use of icebreaker ships, aircraft, and autonomous drones. Establishing a network of supply depots along key routes will ensure the continuous flow of resources.
2. Supply Chain Management: Efficient supply chain management is crucial for the success of the project. Advanced logistics software and real-time tracking systems will be used to monitor and manage the flow of goods and services. Collaboration with international logistics companies will enhance the efficiency and reliability of supply chains.
3. Environmental Conditions: The extreme cold, high winds, and isolation of Antarctica require specialized equipment and infrastructure. This includes insulated habitats, advanced heating systems, and robust communication networks. Continuous environmental monitoring will help anticipate and mitigate the impact of adverse weather conditions.
Economic Challenges
Establishing a sustainable economy in Antarctica is another significant challenge. The success of $PRIM depends on its adoption and use by a wide range of stakeholders.
1. Adoption and Trust: Building trust in $PRIM as a reliable and valuable currency is essential. This will be achieved through transparent governance, robust security measures, and consistent performance. Engaging with the global cryptocurrency community and securing endorsements from reputable figures will also boost confidence in $PRIM.
2. Regulatory Compliance: Ensuring compliance with international laws and regulations is critical. This includes adhering to the principles of the Antarctic Treaty System, which promotes the peaceful and sustainable use of the continent. Legal experts will be consulted to navigate the complex regulatory landscape and ensure that all activities are conducted lawfully.
3. Economic Stability: Maintaining the stability of the $PRIM currency is vital for its success. This will involve implementing monetary policies to manage inflation and prevent excessive volatility. Establishing a reserve fund in traditional currencies or other stable assets will provide a buffer against economic shocks.
Community Building and Social Infrastructure
Establishing a Vibrant Community
Creating a thriving community in Antarctica is key to the long-term success of the Primer Beacon project. This involves attracting a diverse group of individuals and organizations, providing essential services, and fostering a sense of belonging.
1. Attracting Residents: Incentives such as financial rewards, career opportunities, and the unique experience of living in Antarctica will be used to attract residents. Marketing campaigns and partnerships with universities and research institutions will target scientists, engineers, and students interested in contributing to the project.
2. Essential Services: Providing essential services such as healthcare, education, and entertainment is crucial for the well-being of the community. Modern healthcare facilities, remote learning platforms, and recreational activities will be established to ensure a high quality of life for residents.
3. Community Engagement: Fostering a sense of community and belonging is important for social cohesion. Regular community events, social clubs, and collaborative projects will encourage interaction and cooperation among residents. Platforms for communication and feedback will ensure that the needs and concerns of the community are addressed.
Infrastructure Development
Developing robust infrastructure is essential for supporting the Antarctic colony and the Primer Beacon project. This includes housing, transportation, communication, and energy systems.
1. Housing: Modular and prefabricated housing units will be used to provide comfortable and energy-efficient living spaces. These units will be designed to withstand the harsh environmental conditions and can be easily expanded or reconfigured as needed.
2. Transportation: Efficient transportation systems will be established to connect different parts of the colony and facilitate the movement of people and goods. This includes the use of electric vehicles, snowmobiles, and drones. Well-maintained roads and pathways will ensure safe and reliable travel.
3. Communication: Reliable communication networks are vital for coordinating activities and ensuring the safety of residents. Satellite communication systems, high-speed internet, and local networks will provide robust connectivity. Emergency communication protocols will be in place to respond to any incidents.
4. Energy Systems: Sustainable energy systems will be developed to power the colony and the Primer Beacon. This includes the use of solar panels, wind turbines, and advanced energy storage solutions. Smart grid technology will optimize energy distribution and minimize waste.
Environmental Stewardship and Conservation
Minimizing Environmental Impact
The Primer Beacon project is committed to minimizing its environmental impact and preserving the pristine Antarctic environment. This involves implementing sustainable practices and continuously monitoring and mitigating any adverse effects.
1. Sustainable Construction: Sustainable construction practices will be employed to minimize environmental impact. This includes using eco-friendly materials, reducing waste, and implementing energy-efficient designs. Construction activities will be carefully planned and monitored to prevent damage to the local environment.
2. Waste Management: Effective waste management systems will be established to handle all types of waste generated by the colony. This includes recycling, composting, and safe disposal of hazardous materials. Waste reduction strategies, such as minimizing packaging and reusing materials, will also be implemented.
3. Environmental Monitoring: Continuous environmental monitoring will be conducted to assess the impact of the project on the local ecosystem. This includes monitoring air and water quality, wildlife populations, and ice stability. Data from these monitoring efforts will be used to implement mitigation measures and ensure compliance with environmental regulations.
Promoting Conservation and Research
In addition to minimizing its impact, the Primer Beacon project will actively promote conservation and research initiatives to enhance our understanding of the Antarctic environment and contribute to global scientific knowledge.
1. Conservation Initiatives: A portion of $PRIM tokens will be allocated to fund conservation projects in Antarctica. These projects will focus on preserving wildlife, protecting natural habitats, and addressing the impacts of climate change. Collaboration with environmental organizations and research institutions will ensure the effectiveness of these initiatives.
2. Scientific Research: The unique conditions of Antarctica provide valuable opportunities for scientific research. The Primer Beacon project will support research in fields such as glaciology, climatology, biology, and astronomy. Research facilities will be established, and partnerships with universities and research institutions will be fostered.
3. Public Awareness: Raising public awareness about the importance of conservation and the challenges facing the Antarctic environment is crucial. Educational programs, public outreach campaigns, and collaborations with media organizations will be used to disseminate information and engage the global community.
The Antarctic colonization and $PRIM currency initiative represents a bold and innovative approach to addressing the challenges of building and maintaining the Primer Beacon. By creating a sustainable and vibrant community, leveraging advanced technologies, and promoting environmental stewardship, the project aims to achieve its ambitious goals while contributing to global scientific and economic development. The success of this initiative will demonstrate the potential for human ingenuity and collaboration in overcoming the most extreme challenges, paving the way for future exploration and discovery both on Earth and beyond.
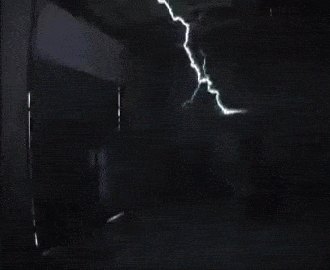
Section 7: Electromagnetic Considerations
Magnetic Field Effects on Human Health
Overview of Antarctic Magnetic Fields
The Earth's magnetic field is a critical factor in maintaining the planet's environment, shielding us from solar radiation and cosmic rays. In Antarctica, the magnetic field is particularly strong, influencing both the environment and any human activities in the region. Understanding the effects of these magnetic fields is essential for the safety and well-being of individuals involved in the Primer Beacon project.
Antarctica's location near the South Magnetic Pole means it experiences stronger and more variable magnetic fields compared to other parts of the world. This has implications for both human health and technological systems. The region's magnetic anomalies can affect everything from navigation and communication systems to the health of those living and working in the area.
Health Impacts of Magnetic Fields
Exposure to strong magnetic fields can have several health effects. While everyday exposure to the Earth's magnetic field is generally considered safe, stronger and more variable fields, such as those found near the poles, can pose risks. Research has indicated that prolonged exposure to intense magnetic fields can lead to a range of health issues, including:
1. Cognitive Effects: Studies have shown that strong magnetic fields can affect cognitive functions. This includes potential impacts on memory, attention, and spatial orientation. The exact mechanisms are not fully understood, but it is believed that magnetic fields can influence the electrical activity of the brain.
2. Sleep Disruption: Magnetic fields can interfere with the body's circadian rhythms, leading to sleep disturbances. This is particularly concerning in environments like Antarctica, where the natural light-dark cycle is already disrupted by extended periods of daylight and darkness.
3. Cardiovascular Effects: There is evidence to suggest that exposure to strong magnetic fields can impact cardiovascular health. This includes changes in heart rate and blood pressure, which could pose risks for individuals with pre-existing health conditions.
4. Other Physiological Effects: Other potential effects include changes in cell function, hormonal balance, and immune response. While the long-term impacts of these changes are not fully understood, they highlight the need for careful monitoring and mitigation strategies.
Mitigation Strategies
To mitigate the potential health effects of exposure to strong magnetic fields in Antarctica, several strategies can be implemented:
1. Shielding: Using materials that can shield against magnetic fields, such as certain metals and alloys, can help protect living and working spaces. Faraday cages, which are enclosures made of conductive materials, can block external electric and magnetic fields, providing a safe environment for residents.
2. Monitoring: Continuous monitoring of magnetic field strength and variations can help identify areas of concern. Portable magnetometers and fixed monitoring stations can be used to track magnetic field exposure in real-time, allowing for timely interventions.
3. Scheduling and Rotation: Implementing work schedules that limit the duration of exposure to strong magnetic fields can reduce health risks. Rotating personnel between high-exposure and low-exposure areas ensures that no individual is subjected to prolonged magnetic field exposure.
4. Research and Education: Ongoing research into the health effects of magnetic fields and the development of best practices for mitigation is essential. Educating personnel about the potential risks and safety measures can also help minimize exposure and prevent adverse health outcomes.
Energy Harvesting Techniques
Utilizing Antarctic Magnetic Fields
The strong magnetic fields in Antarctica can also be harnessed as a source of energy. Electromagnetic induction, the process by which a changing magnetic field induces an electric current in a conductor, can be used to generate electricity. This approach leverages the natural variability and strength of Antarctic magnetic fields to create a sustainable energy source.
1. Magnetic Flux Generators: These devices use the principles of electromagnetic induction to convert magnetic field fluctuations into electrical energy. Placed in areas with strong magnetic field variations, magnetic flux generators can continuously produce electricity. These devices are particularly useful in remote and harsh environments like Antarctica, where traditional energy sources may be limited.
2. Piezoelectric Materials: These materials generate an electric charge in response to mechanical stress. By embedding piezoelectric materials in structures exposed to magnetic field fluctuations, it is possible to convert the mechanical energy from these fluctuations into electrical energy. This method can be integrated into the construction of the Primer Beacon and other infrastructure.
Renewable Energy Integration
In addition to magnetic field-based energy harvesting, integrating other renewable energy sources is crucial for creating a sustainable energy system in Antarctica.
1. Solar Power: Solar panels can capture the abundant sunlight during the Antarctic summer months. Advances in solar technology, such as bifacial panels that capture light from both sides, can maximize energy production. The long daylight hours during summer provide an excellent opportunity for solar energy generation.
2. Wind Power: Wind turbines can harness the strong and consistent winds in Antarctica. Vertical-axis wind turbines, which are more effective in turbulent wind conditions, can provide a reliable source of electricity. These turbines are designed to operate efficiently in extreme cold and high wind speeds.
3. Energy Storage Systems: Effective energy storage solutions, such as lithium-ion batteries and thermal storage, are essential for balancing supply and demand. These systems store excess energy generated during peak production periods and release it when needed, ensuring a continuous power supply.
4. Hybrid Energy Systems: Combining multiple renewable energy sources into a hybrid system enhances reliability and efficiency. Smart grid technology can optimize energy distribution, ensuring that the energy needs of the Primer Beacon and the Antarctic colony are met sustainably.
Future Research Directions
Advanced Energy Harvesting Technologies
Future research will focus on developing and refining advanced energy harvesting technologies that can leverage the unique environmental conditions of Antarctica. This includes:
1. Improving Efficiency: Enhancing the efficiency of magnetic flux generators and piezoelectric materials to maximize energy output. This involves optimizing the design and materials used in these devices.
2. Integration with Infrastructure: Developing methods to seamlessly integrate energy harvesting technologies into the construction of the Primer Beacon and other infrastructure. This includes embedding energy harvesting materials in building materials and structural components.
3. Environmental Impact Assessment: Conducting thorough assessments of the environmental impact of energy harvesting technologies. This ensures that the benefits of these technologies do not come at the expense of the fragile Antarctic ecosystem.
Health and Safety Research
Ongoing research into the health effects of magnetic fields and the development of effective mitigation strategies is essential. This includes:
1. Long-Term Health Studies: Conducting long-term studies on the health effects of magnetic field exposure in Antarctica. This involves monitoring the health of individuals living and working in the region and identifying any correlations between exposure levels and health outcomes.
2. Technological Innovations: Developing new technologies to protect individuals from the adverse effects of magnetic fields. This includes advanced shielding materials and wearable devices that monitor and mitigate exposure.
3. Public Health Guidelines: Establishing clear public health guidelines for safe levels of magnetic field exposure. These guidelines will be based on the latest scientific research and will inform the design and operation of the Antarctic colony.
The successful implementation of the Primer Beacon project in Antarctica requires careful consideration of electromagnetic considerations. By understanding and mitigating the health effects of strong magnetic fields and leveraging these fields for energy harvesting, the project can ensure the safety and sustainability of the Antarctic colony. Ongoing research and technological innovation will play a critical role in addressing these challenges and achieving the project's ambitious goals.
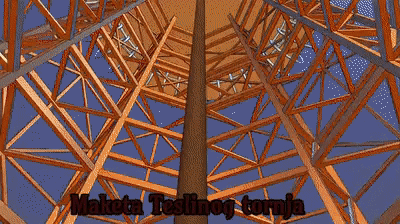
Section 8: Tesla's Wireless Energy Transmission
Historical Background
Nikola Tesla's Vision
Nikola Tesla, one of the most innovative and visionary inventors of the late 19th and early 20th centuries, envisioned a world where electricity could be transmitted wirelessly across vast distances. Tesla's work on wireless energy transmission was rooted in his broader vision of harnessing and distributing electrical power to all parts of the world without the need for traditional transmission lines.
Tesla's most famous project related to wireless energy transmission was the Wardenclyffe Tower, also known as Tesla Tower, constructed in the early 1900s on Long Island, New York. The tower was intended to demonstrate the feasibility of transmitting electrical energy wirelessly using the Earth as a conductor. Although the project was ultimately unsuccessful due to financial difficulties and technical challenges, it laid the groundwork for future research in wireless energy transmission.
The Wardenclyffe Tower
The Wardenclyffe Tower was a 187-foot tall structure topped with a 68-foot diameter dome. Tesla designed the tower to resonate with the natural frequency of the Earth's ionosphere, creating standing waves that could carry electrical energy across great distances. Tesla believed that by using the Earth’s ionosphere, he could transmit electrical power without significant losses, providing free and abundant energy to anyone with a receiver tuned to the correct frequency.
Despite its ambitious goals, the Wardenclyffe Tower project faced numerous obstacles. Financial support for the project dwindled, and Tesla's investor, J.P. Morgan, withdrew his backing. Additionally, the technology of the time was not sufficiently advanced to overcome the practical challenges of wireless energy transmission. In 1917, the tower was demolished, marking the end of Tesla's most ambitious project. However, the concepts and principles Tesla developed have continued to influence modern research in wireless energy transmission.
Modern Applications
Principles of Wireless Energy Transmission
Tesla's principles of wireless energy transmission are still relevant today. The basic concept involves the transmission of electrical energy through the air using electromagnetic waves. There are several methods for achieving this, including:
1. Electromagnetic Induction: This method involves using electromagnetic fields to transfer energy between two coils. The primary coil generates a magnetic field, which induces a current in the secondary coil. This principle is used in wireless chargers for devices like smartphones and electric toothbrushes.
2. Resonant Inductive Coupling: Building on electromagnetic induction, this method involves tuning the coils to resonate at the same frequency, increasing the efficiency of energy transfer over longer distances. Resonant inductive coupling is being explored for wireless charging of electric vehicles and industrial applications.
3. Microwave Transmission: This method involves converting electrical energy into microwaves and transmitting them to a receiver, which converts them back into electrical energy. Microwave transmission is being researched for applications such as space-based solar power, where energy collected by satellites can be beamed back to Earth.
4. Laser Transmission: Similar to microwave transmission, this method uses lasers to transmit energy. The laser beam is directed at a photovoltaic receiver, which converts the light back into electrical energy. Laser transmission is being explored for applications such as powering drones and other remote systems.
Current Technologies and Projects
Several modern projects and technologies are building on Tesla's ideas to develop practical wireless energy transmission systems. These include:
1. WiTricity: A company founded by researchers from the Massachusetts Institute of Technology (MIT), WiTricity focuses on developing wireless charging systems based on resonant inductive coupling. Their technology aims to provide wireless power for electric vehicles, medical devices, and consumer electronics.
2. Space-Based Solar Power: Organizations like the Japan Aerospace Exploration Agency (JAXA) and NASA are researching the feasibility of space-based solar power systems. These systems would collect solar energy in space, where sunlight is constant and unobstructed, and transmit it back to Earth using microwave or laser transmission. This technology has the potential to provide a continuous and renewable source of energy.
3. Dynamic Wireless Charging for Electric Vehicles: Projects such as the European Commission's FABRIC (Feasibility Analysis and Development of On-road Charging Solutions for Future Electric Vehicles) are exploring dynamic wireless charging systems. These systems would allow electric vehicles to charge while driving over specially equipped roads, extending their range and reducing the need for frequent stops.
Potential Benefits and Impact
Enhancing Energy Accessibility
One of the primary benefits of wireless energy transmission is the potential to enhance energy accessibility. By eliminating the need for extensive physical infrastructure, wireless energy systems can bring electricity to remote and underserved areas. This can have a transformative impact on communities that currently lack reliable access to power, improving living standards and enabling economic development.
Wireless energy transmission can also provide a more resilient energy supply. Traditional power grids are vulnerable to natural disasters, technical failures, and cyberattacks. Wireless systems, particularly those using space-based solar power, can offer an independent and reliable energy source that is less susceptible to these risks.
Reducing Environmental Impact
Wireless energy transmission has the potential to reduce the environmental impact of electricity generation and distribution. By enabling more efficient energy transfer, these systems can reduce the losses associated with traditional transmission lines. Additionally, space-based solar power systems can generate clean energy without the land-use and environmental concerns associated with ground-based solar farms and other renewable energy installations.
Furthermore, wireless energy transmission can support the widespread adoption of electric vehicles, reducing reliance on fossil fuels and lowering greenhouse gas emissions. Dynamic wireless charging systems, for example, can make electric vehicles more convenient and practical, encouraging more people to make the switch from gasoline-powered cars.
Challenges and Future Research Directions
Technical Challenges
Despite the promising potential of wireless energy transmission, several technical challenges must be addressed to make it a viable alternative to traditional systems. These include:
1. Efficiency: Current wireless energy transmission methods still face significant efficiency losses, particularly over long distances. Research is needed to develop more efficient transmission technologies and to minimize energy losses during conversion and transmission.
2. Safety: Ensuring the safety of wireless energy transmission systems is crucial. High-power microwave and laser transmission can pose risks to humans, animals, and electronic devices. Developing safety protocols and technologies to mitigate these risks is essential for the widespread adoption of wireless energy systems.
3. Regulation and Standardization: Establishing regulatory frameworks and industry standards for wireless energy transmission is necessary to ensure compatibility, safety, and reliability. International cooperation will be required to develop and implement these standards.
Future Research Directions
Future research in wireless energy transmission will focus on addressing these technical challenges and exploring new applications. Key areas of research include:
1. Advanced Materials: Developing new materials with enhanced electrical and magnetic properties can improve the efficiency and effectiveness of wireless energy transmission systems. This includes materials for more efficient antennas, receivers, and energy storage systems.
2. Integration with Renewable Energy: Researching ways to integrate wireless energy transmission with renewable energy sources, such as solar and wind power, can create more sustainable and resilient energy systems. This includes developing hybrid systems that combine different transmission methods and energy sources.
3. Space-Based Applications: Continued exploration of space-based applications, such as solar power satellites and wireless power for space missions, will expand the potential uses of wireless energy transmission. This research can also contribute to the development of technologies for long-distance energy transmission on Earth.
4. Interdisciplinary Collaboration: Collaboration between scientists, engineers, policymakers, and industry leaders will be essential for advancing wireless energy transmission technologies. Interdisciplinary research can address technical, regulatory, and social challenges, paving the way for the successful implementation of these systems.
Tesla's vision of wireless energy transmission continues to inspire modern research and innovation. By leveraging advanced technologies and addressing key challenges, we can develop practical and efficient wireless energy systems that enhance energy accessibility, reduce environmental impact, and support sustainable development. As research progresses, the principles and ideas pioneered by Tesla will remain a guiding force in the quest to harness and distribute electrical power without the constraints of traditional transmission methods.
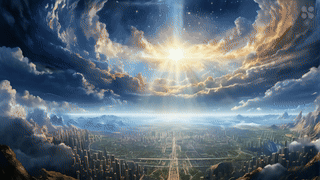
Section 9: Extraterrestrial Life & Genetic Parallels
Historical Encounters
Ancient Civilizations and Alien Encounters
Throughout history, numerous ancient civilizations have recorded encounters with beings that they described as gods, angels, or visitors from the sky. These accounts, found in various cultural myths, religious texts, and historical records, often describe interactions with advanced beings who possessed extraordinary knowledge and technology. While these stories are often interpreted metaphorically or mythologically, some researchers propose that they could represent actual encounters with extraterrestrial beings.
-
Sumerian Civilization: One of the earliest known civilizations, the Sumerians, recorded their interactions with the Anunnaki, a group of deities who were believed to have come from the sky. According to Sumerian texts, the Anunnaki were responsible for the creation of humanity and imparted advanced knowledge to the Sumerians.
-
Egyptian Civilization: Ancient Egyptian texts and hieroglyphs also contain references to beings from the stars. The gods of ancient Egypt, such as Ra, Osiris, and Thoth, were often depicted as having celestial origins. The construction of the pyramids and other monumental structures has led to speculation that extraterrestrial beings may have assisted or guided the Egyptians.
-
Mesoamerican Civilizations: The Mayans and Aztecs, among other Mesoamerican cultures, also recorded encounters with gods who descended from the heavens. Quetzalcoatl, a prominent deity in Mesoamerican mythology, is described as a feathered serpent who brought knowledge and civilization to the people. Some researchers suggest that these descriptions could be based on interactions with extraterrestrial beings.
-
Indian Civilization: Ancient Indian texts, such as the Vedas and the Mahabharata, contain detailed descriptions of flying machines called vimanas and battles between gods that took place in the sky. These accounts, which include references to advanced technology and powerful weapons, have been interpreted by some as evidence of extraterrestrial encounters.
Modern UFO Sightings and Alien Abductions
In addition to historical accounts, modern reports of unidentified flying objects (UFOs) and alien abductions have contributed to the ongoing debate about extraterrestrial life. While many of these reports can be explained by natural phenomena or human-made objects, some remain unexplained and continue to intrigue researchers and the public.
-
Roswell Incident (1947): One of the most famous UFO incidents occurred in Roswell, New Mexico, where a purported alien spacecraft crashed. The U.S. military initially reported recovering a "flying disc," but later retracted the statement, claiming it was a weather balloon. The incident has sparked numerous conspiracy theories and remains a focal point for UFO enthusiasts.
-
Betty and Barney Hill Abduction (1961): The case of Betty and Barney Hill is one of the earliest and most well-documented alien abduction stories. The couple claimed to have been taken aboard an alien spacecraft and subjected to medical examinations. Their account, corroborated by physical evidence and hypnosis sessions, has been extensively studied and debated.
-
Phoenix Lights (1997): Thousands of people in Phoenix, Arizona, reported seeing a series of lights in a V-shaped formation moving across the sky. The incident, witnessed by residents and documented by media, remains unexplained despite various official explanations. The Phoenix Lights are considered one of the most credible mass UFO sightings.
-
Navy Pilots' Encounters (2004 and 2015): Recently declassified footage from U.S. Navy pilots shows encounters with unidentified aerial phenomena (UAPs) displaying extraordinary flight capabilities. These encounters, recorded on radar and infrared cameras, have been acknowledged by the Pentagon and have reignited interest in the search for extraterrestrial life.
Biological Implications
Panspermia Hypothesis
The panspermia hypothesis suggests that life on Earth may have originated from microorganisms or chemical precursors of life present in outer space and brought to Earth via comets, asteroids, or interstellar dust. This hypothesis posits that life is not confined to Earth and could be widespread throughout the universe.
-
Meteorite Evidence: Certain meteorites, such as the Murchison meteorite, have been found to contain organic compounds, including amino acids, which are the building blocks of life. This supports the idea that the basic ingredients for life could be distributed throughout the cosmos.
-
Survivability of Microorganisms: Experiments have shown that certain microorganisms can survive the harsh conditions of space, including extreme temperatures, radiation, and vacuum. This suggests that microbial life could potentially travel between planets and even star systems, seeding life wherever conditions are favorable.
-
Interplanetary Transfer: The concept of lithopanspermia suggests that rocks ejected from a planet by impacts could carry microorganisms to another planet. This process could explain how life might spread within a solar system. For example, it is hypothesized that life could have been transferred between Mars and Earth via meteorites.
Genetic Parallels
The search for extraterrestrial life also includes the study of potential genetic parallels between Earth life and hypothetical alien life forms. Scientists explore the possibility that if life exists elsewhere, it might share some fundamental biochemical properties with life on Earth.
-
Universal Biochemistry: The basic building blocks of life on Earth, such as DNA, RNA, and proteins, might also be found in extraterrestrial organisms. The universality of these molecules in Earth's biochemistry suggests that they could be a common template for life throughout the universe.
-
Alternative Biochemistries: Researchers also consider the possibility of alternative biochemistries that differ from those found on Earth. For example, life could be based on silicon instead of carbon, or use different solvents like methane or ammonia instead of water. Studying these possibilities expands our understanding of what life might look like on other planets.
-
Astrobiology and Exoplanets: The discovery of exoplanets in the habitable zones of their stars has intensified the search for extraterrestrial life. Astrobiologists study these planets' atmospheres, climates, and potential biosignatures to identify signs of life. The detection of molecules like oxygen, methane, or water vapor in an exoplanet's atmosphere could indicate biological processes similar to those on Earth.
The study of extraterrestrial life and genetic parallels encompasses a wide range of disciplines, including history, biology, astronomy, and genetics. By examining historical encounters, modern UFO sightings, and potential genetic parallels, researchers are expanding our understanding of the possibilities for life beyond Earth. Ongoing research in astrobiology, genetic studies, and synthetic biology continues to push the boundaries of our knowledge.
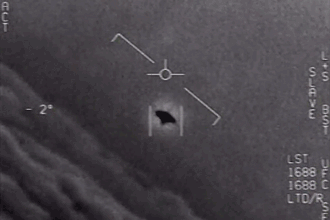
Section 10: Terraforming Venus
Current Status
Venusian Environment
Venus, often referred to as Earth's "sister planet," presents one of the most challenging environments in our solar system. With surface temperatures exceeding 460 degrees Celsius (860 degrees Fahrenheit) and atmospheric pressure about 92 times that of Earth, Venus's environment is harsh and hostile to life as we know it. The thick atmosphere, composed mainly of carbon dioxide with clouds of sulfuric acid, creates a runaway greenhouse effect, making the surface incredibly hot and inhospitable.
The planet’s slow rotation results in a day-night cycle longer than its year, and its lack of a significant magnetic field exposes the surface to solar and cosmic radiation. Despite these extreme conditions, the possibility of terraforming Venus remains a topic of interest due to its proximity to Earth and its similarities in size and composition.
Exploration Missions
Various missions have been sent to Venus to study its atmosphere and surface conditions. The Soviet Venera program in the 1960s and 1970s successfully landed probes on the surface, providing the first images and data from the planet’s surface. NASA's Magellan mission in the early 1990s mapped the surface using radar, revealing a landscape dominated by volcanic plains, mountains, and vast lava flows.
More recent missions, such as the European Space Agency's (ESA) Venus Express (2005-2014) and Japan's Akatsuki mission, have focused on atmospheric studies. These missions have provided valuable insights into the dynamics of Venus's atmosphere, its chemical composition, and the nature of its clouds.
Future Prospects
Terraforming Strategies
Terraforming Venus involves altering its environment to make it more Earth-like, potentially supporting human life. Several strategies have been proposed, each addressing the planet's extreme conditions in different ways:
1. Atmospheric Cooling: One approach involves cooling Venus’s atmosphere to reduce temperatures and condense the carbon dioxide. This could potentially be achieved using large space-based reflectors or shades to block sunlight from reaching the planet. By reducing the influx of solar energy, the planet’s surface temperature could be lowered over time.
2. Carbon Dioxide Removal: Another strategy focuses on removing or converting the thick carbon dioxide atmosphere. One proposal is to use photosynthetic microorganisms or chemical processes to convert CO2 into oxygen and other compounds. Introducing genetically engineered bacteria that can survive in extreme conditions and perform photosynthesis might gradually transform the atmosphere.
3. Introducing Water: Water is crucial for life, and introducing it to Venus could help in terraforming. This could involve redirecting comets or ice-rich asteroids to collide with Venus, delivering water to the surface. Additionally, if bacteria in the clouds, 50 km above the surface where the temperature is a pleasant 25 degrees Celsius, can reduce atmospheric pressure and temperature by extracting carbon from the atmosphere, the water vapor produced by their biochemical processes could eventually condense to form oceans and lakes over eons.
4. Rotational Adjustment: Adjusting Venus's slow rotation might help in creating a more Earth-like day-night cycle. This could theoretically be achieved through massive space-based structures or interactions with large celestial bodies. However, this approach is highly speculative and presents significant technical challenges.
Ethical and Environmental Considerations
Terraforming Venus also raises significant ethical and environmental questions. Altering a planet’s environment on such a massive scale could have unforeseen consequences for the planet itself and the broader solar system.
1. Planetary Protection: The concept of planetary protection involves preserving other worlds in their natural state to avoid contaminating them with Earth life or altering their environments. Terraforming efforts could conflict with these principles, raising questions about our right to alter another planet.
2. Unintended Consequences: Large-scale environmental modifications could lead to unintended and potentially harmful consequences. For example, the introduction of water and microbial life could trigger unexpected chemical reactions or ecological imbalances, leading to unpredictable outcomes.
3. Ethical Considerations: The ethical implications of terraforming involve considering the potential benefits and harms to future generations, both on Earth and Venus. The resources required for such an endeavor could be immense, and the potential benefits need to be weighed against other pressing needs on Earth, such as addressing climate change and sustainable development.
Technological Developments
Advances in technology are essential for the feasibility of terraforming Venus. Current research focuses on developing the tools and techniques needed for such an ambitious project.
1. Advanced Materials: Developing materials that can withstand the extreme conditions on Venus is crucial. This includes materials for constructing space-based reflectors, habitats, and other infrastructure needed for terraforming efforts.
2. Energy Solutions: Efficient and sustainable energy solutions are required to power terraforming activities. This includes solar power, nuclear energy, and other advanced energy technologies that can operate in the harsh Venusian environment.
3. Robotic and Autonomous Systems: Robotic systems and autonomous vehicles will play a key role in terraforming efforts. These technologies can perform tasks in environments that are too hostile for humans, such as constructing infrastructure, deploying reflectors, and managing biological processes.
Terraforming Venus represents one of the most ambitious and challenging projects ever conceived. While current technology and understanding are not yet sufficient to undertake such an endeavor, ongoing research in astrobiology, extremophiles, and advanced materials continues to push the boundaries of what is possible. The potential benefits of terraforming, such as creating a new habitat for humanity and expanding our presence in the solar system, must be carefully weighed against the significant ethical, environmental, and logistical challenges. As we continue to explore and understand our neighboring planets, the dream of transforming Venus into a more Earth-like world remains a compelling vision for the future.
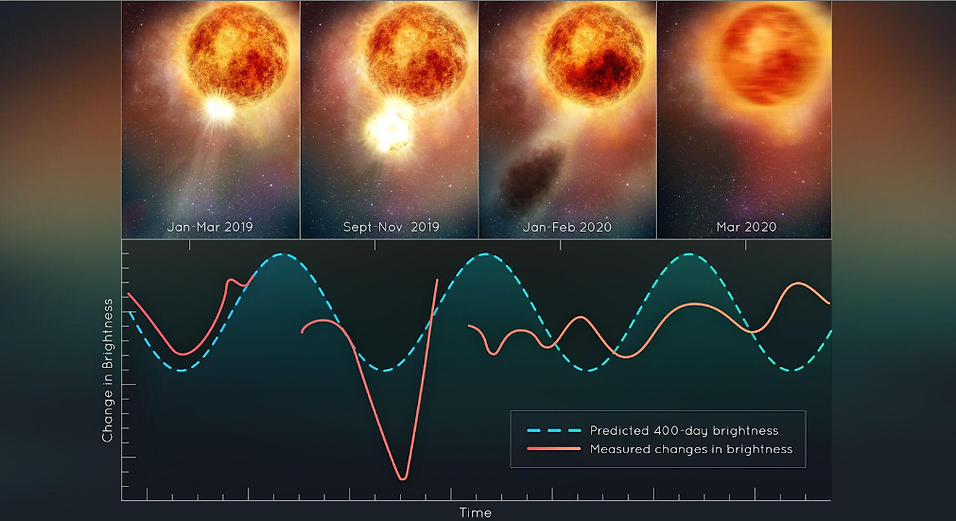
Section 11: Planetary Formation
Decay Model of Planetary Formation
Historical Perspectives
The study of planetary formation has evolved significantly over the past few centuries. Historically, the predominant model was the nebular hypothesis, first proposed by Immanuel Kant and further developed by Pierre-Simon Laplace in the 18th century. This model suggests that planets form from the gravitational collapse of a cloud of gas and dust in a protoplanetary disk around a new star. According to this hypothesis, the outer regions of the disk, where temperatures are lower, give rise to gas giants, while the inner regions form rocky planets.
However, modern observations and discoveries have challenged this classical view. The presence of "hot Jupiters," gas giants located very close to their parent stars, contradicts the traditional nebular hypothesis. These observations have prompted scientists to explore alternative models, such as the core accretion model and the disk instability model, to explain the diversity of planetary systems observed in our galaxy.
The Decay Model
The decay model of planetary formation draws an analogy between planetary systems and atomic structures, particularly focusing on decay events within an atom. In atomic physics, alpha decay involves the ejection of a helium nucleus, while beta decay involves the transformation of a neutron into a proton and the emission of an electron. These processes release significant energy and result in changes to the nucleus.
Applying this analogy to planetary systems, the decay model suggests that planets could form through a process similar to nuclear decay. For instance, a star might eject a proto-planetary body in a manner analogous to alpha decay. This body would then accrete material and potentially form a new planet. This model emphasizes the dynamic and potentially violent processes involved in planetary formation and evolution, including interactions with the star's magnetic field and gravitational perturbations from other bodies in the system.
Future Research Directions
Advanced Observational Techniques
Future research will continue to leverage advanced observational techniques to study planetary formation and search for habitable exoplanets.
-
Direct Imaging: New telescopes equipped with advanced coronagraphs and starshades will improve our ability to directly image exoplanets, allowing for detailed study of their atmospheres and surfaces.
-
Spectroscopy: High-resolution spectroscopy will enable the detection of a wide range of chemical compounds in exoplanet atmospheres, providing insights into their composition and potential biosignatures.
-
Astrometric Measurements: Precise measurements of a star’s position and motion, known as astrometry, can reveal the presence of exoplanets and provide data on their orbits and masses. This information is crucial for understanding the dynamics of planetary systems.
-
Fast Radio Bursts & New Planets: If the creation of planets is in accordance with the Decay Model one might observe in the night sky an event like the gamma-ray burst in the atom which accompanies Alpha and Beta decay events. Could it be the attendant radiation in the case of planets birthing is none other than the all-too-common phenomenon known as the Fast Radio Burst? If one indexed each star and its exoplanets then watched all the stars for FRBs one could check to see if the FRB source matched with a star which has just gained a new planet via such techniques as the Transit method.
The study of planetary formation is a rapidly advancing field that combines observational astronomy, theoretical modeling, and interdisciplinary research.
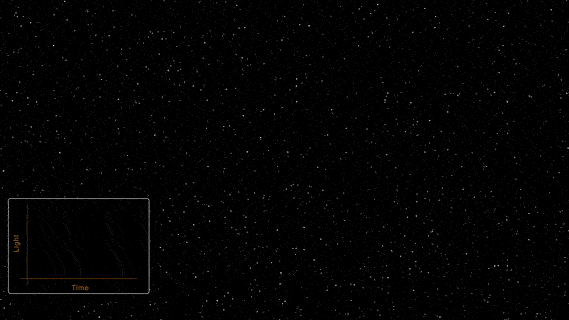
Section 12: Biblical Prophecies and Modern Science
Interpretations of Ancient Texts
The Bible and Extraterrestrial Life
The Bible has been a source of inspiration and guidance for billions of people over the millennia. Within its texts, some interpretations suggest references to extraterrestrial beings and advanced technologies. Scholars and theologians have debated these interpretations, with some proposing that ancient texts contain veiled descriptions of encounters with advanced beings or technologies that were beyond the understanding of the people at the time.
1. Ezekiel’s Vision: One of the most frequently cited passages in discussions of extraterrestrial life is the vision of Ezekiel. In the Book of Ezekiel, the prophet describes a vision of a "wheel within a wheel" in the sky, accompanied by beings with a human-like appearance but with extraordinary features. Some researchers suggest that this description could be interpreted as a sighting of an advanced spacecraft and its occupants.
2. Genesis 6:1-4: The passage in Genesis that describes the "sons of God" coming down to Earth and marrying the "daughters of men" has been interpreted by some as a reference to extraterrestrial beings interacting with humans. The offspring of these unions, described as "giants" or "Nephilim," are seen by some as evidence of genetic manipulation or hybridization between humans and extraterrestrial beings.
3. The Book of Revelation: The apocalyptic imagery in the Book of Revelation includes descriptions of heavenly battles, advanced weapons, and celestial phenomena. Some interpreters suggest that these visions might describe future events involving extraterrestrial civilizations or advanced technologies that were perceived in symbolic terms by the ancient author.
Modern Science and Biblical Prophecies
Technological Interpretations
Modern science and technology offer new ways to interpret biblical prophecies. Advances in space exploration, genetics, and artificial intelligence provide frameworks for understanding ancient texts in the context of potential encounters with advanced civilizations.
1. Space Exploration: The vision of Ezekiel and other biblical descriptions of celestial phenomena can be reinterpreted through the lens of modern space exploration. For example, the "wheel within a wheel" could be seen as a description of a spacecraft, while other visions might describe interactions with robotic probes or other advanced technologies.
2. Genetic Engineering: The story of the Nephilim in Genesis could be viewed in light of modern genetic engineering. The idea of "sons of God" engaging with humans might parallel contemporary discussions about the potential for creating human-animal hybrids or using advanced genetic techniques to alter the human genome.
3. Artificial Intelligence: Prophecies involving advanced knowledge and wisdom could be interpreted as references to artificial intelligence. The development of AI and its potential to surpass human intelligence aligns with descriptions of beings with extraordinary capabilities and knowledge.
4. Common Origin Theory: One explanation for how beings from the sky interbred with humans on Earth is that we share the same DNA. This suggests a common origin. Perhaps the larger picture involves the growth and colonization of other worlds via a much longer plan - from archaea to man in a number of preplanned steps hidden within the massive code each organism capable of taking space flight from Earth to space potentially contains.
Future Predictions
Space Colonization and Biblical Prophecies
The Bible contains numerous prophecies about the future, some of which can be interpreted as foretelling the expansion of humanity into space. Modern efforts to colonize the Moon, Mars, and beyond resonate with these ancient predictions, suggesting that humanity's destiny may indeed lie among the stars.
1. The New Jerusalem: The Book of Revelation describes the descent of the New Jerusalem from heaven, a vision that some interpret as a reference to a space station or a colony descending from space. This imagery aligns with contemporary efforts to build permanent habitats in space, such as lunar bases and Martian colonies.
2. Inheriting the Stars: Biblical passages that speak of humanity inheriting the Earth and the heavens can be seen as prophetic of our potential future as a spacefaring species. As we develop the technology to explore and colonize other planets, these ancient prophecies gain new significance.
Ethical and Theological Considerations
The potential discovery of extraterrestrial life and the expansion of humanity into space raise significant ethical and theological questions. How we reconcile these developments with biblical teachings will shape our approach to space exploration and our understanding of humanity's place in the cosmos.
1. Theological Implications: The discovery of extraterrestrial life would challenge many traditional theological concepts, prompting a re-evaluation of doctrines related to creation, salvation, and the uniqueness of humanity. Theologians would need to consider how extraterrestrial beings fit into the biblical narrative and what their existence means for our understanding of God and creation.
2. Ethical Considerations: The ethical implications of space colonization, such as the treatment of any indigenous life forms we might encounter, the preservation of planetary environments, and the equitable distribution of space resources, must be addressed. These considerations align with biblical principles of stewardship, justice, and compassion.
Integrating Science and Faith
Dialogue Between Science and Religion
The intersection of biblical prophecies and modern science provides a unique opportunity for dialogue between science and religion. By exploring these connections, we can foster a deeper understanding of both realms and find common ground in our quest for knowledge and meaning.
1. Interdisciplinary Research: Encouraging interdisciplinary research that includes theologians, scientists, and ethicists can lead to a more comprehensive understanding of the implications of space exploration and the potential discovery of extraterrestrial life. This collaborative approach can help bridge the gap between scientific and religious perspectives.
2. Public Engagement: Engaging the public in discussions about the relationship between biblical prophecies and modern science can promote greater awareness and understanding of these complex issues. Educational programs, public lectures, and media initiatives can facilitate informed dialogue and encourage critical thinking.
The study of biblical prophecies in the context of modern science offers a fascinating and thought-provoking exploration of humanity's past, present, and future. By examining ancient texts through the lens of contemporary technology and scientific understanding, we can uncover new insights and perspectives on our place in the universe. As we continue to explore the cosmos and push the boundaries of knowledge, the dialogue between science and religion will remain essential in shaping our vision for the future and guiding our actions in the pursuit of discovery and understanding.
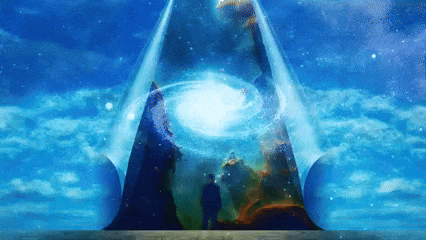
Section 13: Tether the Moon
Further Explorations
In the future it might be feasible to remove the ice from the Antarctic without melting it or causing a 60-m rise in oceans worldwide. For example, we could port it to the moon, for use by a moon colony, making the water problem a clean win, in addition to creating a major improvement for the Beacon to anchor down without having to drill through kilometers of ice. Transporting ice from Antarctica to the Moon involves multiple complex steps, including extraction, transportation to the lunar tether, transfer to the Moon, and final processing into water. Here is a detailed plan:
Extraction and Initial Transport
-
Extraction of Ice:
- Location Selection: Identify the most feasible areas in Antarctica for ice extraction, focusing on regions with thick, stable ice sheets.
- Mining Operations: Use large-scale ice mining equipment to cut and collect ice blocks. Consider environmentally friendly and sustainable methods to minimize ecological impact.
2. Initial Transport:
- Conveyor Systems: Set up conveyor belts or pipeline systems to transport ice from the mining site to the coast.
- Ice Transport Ships: Utilize specialized ships designed for carrying large quantities of ice. These ships should be equipped with refrigeration systems to prevent melting during transport.
Transport to the Lunar Tether
-
Transfer to Launch Sites:
- Port Facilities: Establish port facilities with the infrastructure to handle large ice shipments.
- Loading: Load ice onto craft capable of transporting large masses.
2. Transport:
- Cargo Vehicles: Use heavy-lift vehicles to transport ice to a staging point.
- Transfer Vehicles: Employ transfer vehicles to move the ice to the tether point.
Transfer to the Lunar Surface
1. Tether Climber System:
- Loading at the Bob: Use robotic systems to transfer ice from transfer vehicles to the tether climber.
- Tether Climber: Design a climber capable of carrying large ice blocks. Ensure the climber has sufficient power and structural integrity to handle the weight and maintain stability during transit.
2. Lunar Descent:
- Controlled Descent: The climber descends along the tether to the lunar surface, using regenerative braking to manage speed and recapture energy.
Processing on the Moon
-
Lunar Ice Processing Facility:
- Unloading: Establish facilities to unload and store ice on the Moon.
- Melting and Purification: Set up systems to melt the ice and purify it for use as water. This includes filtration, sterilization, and potentially electrolysis to separate water into hydrogen and oxygen for various uses.
Engineering and Logistical Considerations
1. Material Requirements:
- High-Strength Materials: Use materials like carbon nanotubes for the tether to ensure it can support the weight and stress of continuous ice transport.
- Thermal Management: Incorporate insulation and cooling systems to prevent ice from melting during transport.
2. Environmental and Ethical Considerations:
- Sustainability: Ensure that ice extraction does not significantly impact the Antarctic ecosystem.
- International Regulations: Comply with international treaties and regulations regarding Antarctic resource extraction and space operations.
Implementation Phases
1. Feasibility Study: Conduct comprehensive studies to assess the technical, environmental, and economic feasibility of the project.
2. Prototype Development: Develop and test prototypes for each phase of the transport process.
3. Pilot Project: Implement a small-scale pilot project to test the entire transport chain from Antarctica to the Moon.
4. Full-Scale Operation: Gradually scale up operations, incorporating lessons learned from the pilot project.
Transporting ice from Antarctica to the Moon using a tether system involves sophisticated engineering and logistics, from ice extraction and transport to transfer to the tether, and finally processing on the lunar surface. Each step requires careful planning, innovative technology, and adherence to environmental and ethical standards to ensure the success and sustainability of the project.
Moon Tether
To use the lunar tether system for transporting objects between the Moon and a point near Earth, you would need a well-designed transportation mechanism that can move along the tether. Here's a detailed plan on how to achieve this:
Transportation Mechanism
1. Design of the Transport System:
- Tether Climber: The primary transport mechanism would be a tether climber, a vehicle designed to ascend and descend the tether.
- Wheels or Tracks: The climber could use wheels or tracks that grip the tether, allowing it to move smoothly up and down.
- Power Source: Power could be provided through solar panels, batteries, or beamed energy (microwave or laser). Another possibility is to anchor the tether to the South Pole upon a rotating shaft in the ground, which can generate enough electricity to power not only the tether but the regions surrounding the shaft for some distance.
- Payload Module: An interchangeable payload module for transporting cargo or passengers.
2. Components of the Tether Climber:
- Drive System: Motors that drive the wheels or tracks.
- Energy System: Solar panels for power during the journey, supplemented by batteries and the tether shaft generator.
- Control System: Automated guidance and control system to ensure stability and safety.
Operation of the Transport System
-
Starting from the Moon:
- Loading Phase: Load cargo or passengers onto the tether climber at the lunar base.
- Initial Ascent: The climber ascends the tether using its drive system, powered by solar panels and batteries. Current from the shaft generator at Earth’s South Pole may also be drawn via cables in the tether.
- Mid-Transit Phase: During transit, the climber will continuously adjust its position to maintain stability.
2. Reaching the South Pole of Earth:
- Stop and Transfer: The climber reaches the shaft anchoring the tether at Earth’s South Pole.
- Loading/Unloading: Cargo or passengers can be transferred to another vehicle if needed for further transit to Earth destinations.
3. Ascent to the Moon:
- Return Trip: After unloading, the climber ascends back to the Moon, powered by the tether shaft generator and solar panels.
Detailed Engineering Considerations
1. Tether Climber Specifications:
- Weight and Balance: Must be balanced to avoid oscillations or destabilizing the tether.
- Gripping Mechanism: Strong enough to handle the tether's material and withstand the forces during transit.
- Safety Systems: Redundant systems for braking, emergency power, and communication.
2. Energy and Propulsion:
- Energy Efficiency: Use of regenerative braking to recapture energy during descent. Wires in the tether carry current generated at Earth's South Pole by the rotating shaft the tether is linked with.
- Power Management: Intelligent systems to manage power usage based on shaft current, solar availability and battery levels.
Maintenance and Monitoring
1. Tether Maintenance:
- Regular Inspections: Automated drones or climbers equipped with sensors to inspect and repair the tether.
- Repair Kits: Climber modules equipped with repair tools and materials.
2. System Monitoring:
- Control Center: Centralized control center on the Moon for monitoring and controlling climber operations.
- Telemetry: Real-time data transmission to monitor the health of the tether and climber.
Applications
-
Scientific Research:
- Transporting scientific instruments and samples between the Moon and the South Pole.
2. Commercial Use:
- Moving commercial cargo, such as mined lunar resources, to Earth.
3. Human Transportation:
- Safe transport of astronauts and personnel between lunar bases and Earth.
Implementation Steps
1. Feasibility Studies: Conduct detailed studies to refine the design and address potential issues.
2. Prototype Development: Develop and test prototypes of the tether climber in controlled environments.
3. Pilot Projects: Implement pilot projects to test the system in lunar conditions.
4. Full Deployment: Gradually scale up the system for full operational capability.
By following these steps and considerations, a lunar tether system can be effectively utilized to transport objects between the Moon and a point near Earth, leveraging the innovative tether technology and ensuring efficient and safe operations.
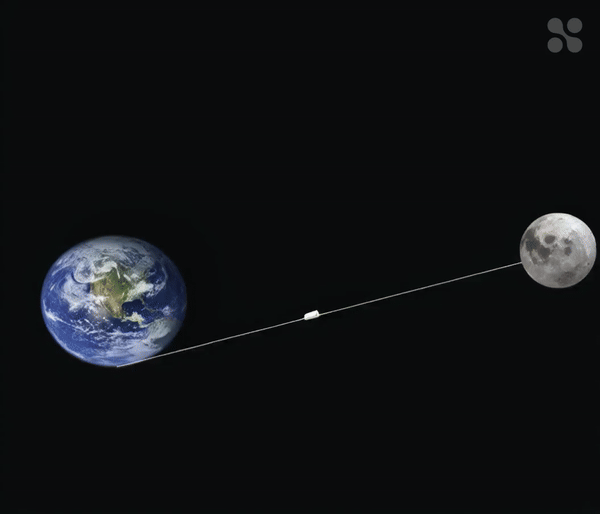
Tether the South Pole
Generator!
Connecting the Earth's south pole to a point on the Moon involves selecting an optimal anchoring spot on the Moon that maximizes the feasibility and utility of the tether system. Given the Moon's tidal locking, it always shows the same face towards Earth, which simplifies the selection process.
Optimal Anchoring Spot on the Moon
Considerations for Selection
1. Tidal Locking: The Moon's near side is constantly facing the Earth, making it the logical area to consider for the tether's anchor point.
2. Stability and Accessibility: The site should be geologically stable and accessible for construction and maintenance.
3. Location Relative to Earth’s South Pole: The anchoring point should ideally be directly in the line of sight of the Earth's south pole to minimize the tether's length and optimize alignment.
Calculations and Geometric Considerations
Geostationary-like Positioning on the Moon
The Moon doesn't have geostationary positions like satellites in Earth's orbit, but certain points on the near side are always visible from Earth due to tidal locking. We need to find a point that would be directly above the Earth's south pole during the Moon's orbit around Earth.
1. Simplified Alignment:
- The Moon's distance from Earth: (approx 384,400 km)
- The Earth’s axial tilt affects where the tether will connect on the Moon’s surface.
Potential Anchoring Points
1. Equatorial Regions:
- The equatorial regions of the near side of the Moon are always facing Earth and provide a direct line of sight. This simplifies the tether's deployment.
- Optimal equatorial coordinates would be around 0^ latitude and 0^ to 5^ longitude (near the Moon's prime meridian).
2. Highland Regions:
- The lunar highlands, specifically near the equatorial region, offer stable ground for anchoring.
- Montes Apenninus or the region around Mare Imbrium is a potential site due to its relatively stable surface and proximity to the equator.
Advantages of the Proposed Site
1. Direct Visibility: Ensures continuous visibility and alignment with Earth’s south pole.
2. Stable Surface: Highlands provide a more solid foundation for anchoring heavy structures.
3. Energy Generation Potential: Direct tethering allows for the transfer of rotational energy from Earth's rotation to the tether system, maximizing energy generation.
Final Selection
Based on the analysis, the optimal point on the Moon for anchoring the tether would be:
Coordinates: Near 0^ latitude and 0^ to 5^ longitude, around the Montes Apenninus or Mare Imbrium region.
Conclusion
By anchoring the tether at this optimal point, you can maximize the stability and efficiency of the tether system, potentially generating significant amounts of electricity to support the colonization of the Earth's south pole. This location offers direct visibility, a stable surface, and favorable geometric alignment with the Earth’s south pole.
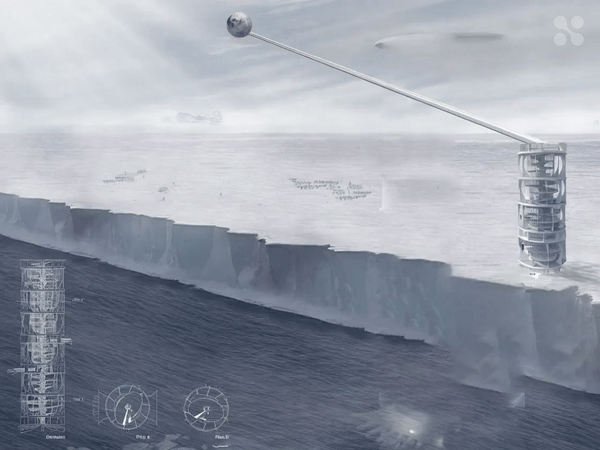
Section 14: Recommendations
Strategic Objectives
Enhancing Research and Exploration
To advance our understanding of extraterrestrial life and planetary science, we must prioritize enhancing our research and exploration capabilities. This involves investing in cutting-edge technologies, fostering international collaboration, and supporting interdisciplinary research. Key objectives include:
1. Developing Advanced Spacecraft: Investing in the development of next-generation spacecraft with advanced observational instruments will enable more detailed studies of exoplanets, the polar regions of the gas giants, and other celestial phenomena. These spacecraft should be equipped with high-resolution spectrometers, powerful telescopes, and robust communication systems.
2. Expanding Space Missions: Increasing the number and scope of space missions to explore planets, moons, and other bodies within our solar system is crucial. Missions such as NASA’s Europa Clipper, the European Space Agency’s JUICE mission, and future missions to the outer planets will provide valuable data on the potential for life and the processes of planetary formation.
3. Promoting International Collaboration: Encouraging collaboration between space agencies, research institutions, and private companies will leverage diverse expertise and resources. International partnerships, such as those seen in the International Space Station (ISS) and the Artemis program, can accelerate progress and share the benefits of exploration.
4. Supporting Interdisciplinary Research: Fostering interdisciplinary research that integrates astronomy, biology, geology, and other sciences will provide a holistic understanding of extraterrestrial environments and the potential for life. Initiatives like NASA’s Astrobiology Institute and the European Astrobiology Network Association (EANA) exemplify the benefits of interdisciplinary collaboration.
Technological Innovation and Development
Advancing Key Technologies
Developing and deploying new technologies is essential for the success of future exploration missions and the study of extraterrestrial life. Key areas for technological innovation include:
1. High-Resolution Imaging: Developing advanced imaging technologies, such as space-based interferometers and adaptive optics systems, will improve our ability to detect and study distant exoplanets and their atmospheres. These technologies can reveal detailed information about planetary surfaces, weather patterns, and potential biosignatures.
2. Autonomous Systems and AI: Utilizing autonomous systems and artificial intelligence (AI) in space missions can enhance data collection, analysis, and decision-making. AI-driven rovers, probes, and satellites can operate in harsh environments, conduct complex experiments, and transmit critical data back to Earth in real-time.
3. Renewable Energy Solutions: Implementing renewable energy solutions, such as solar and wind power, for space missions and extraterrestrial colonies will ensure sustainable energy supply. Developing efficient energy storage systems and wireless energy transmission technologies will support long-term exploration and habitation.
4. Biotechnology and Synthetic Biology: Advancing biotechnology and synthetic biology will enable the creation of organisms and materials tailored for extraterrestrial environments. These innovations can support life support systems, resource extraction, and environmental management in space colonies.
Ethical and Environmental Stewardship
Ensuring Responsible Exploration
The exploration and potential colonization of other planets must be conducted responsibly, with careful consideration of ethical principles and environmental impacts. Recommendations for ensuring responsible exploration include:
1. Adhering to Planetary Protection Protocols: Following planetary protection protocols, as outlined by the Committee on Space Research (COSPAR), will prevent biological contamination of other planets and protect Earth from potential extraterrestrial organisms. These protocols are essential for preserving the integrity of scientific research and protecting planetary environments.
2. Implementing Sustainable Practices: Employing sustainable practices in space missions and extraterrestrial colonies will minimize environmental impact and promote long-term viability. This includes using renewable energy, recycling resources, and minimizing waste generation.
3. Addressing Ethical Implications: Engaging ethicists, theologians, and other experts in discussions about the ethical implications of space exploration will ensure that diverse perspectives are considered. This includes addressing questions about the rights of potential extraterrestrial life forms, the preservation of pristine environments, and the fair distribution of space resources.
4. Promoting Public Engagement: Involving the public in discussions about space exploration and its ethical considerations will foster greater awareness and understanding. Educational programs, public lectures, and media initiatives can encourage informed dialogue and support for responsible exploration practices.
Implementation Plan
Short-Term Actions
1. Launch Research Initiatives: Establish new research initiatives focused on advanced imaging, AI, renewable energy, and biotechnology. Secure funding and partnerships with academic institutions, private companies, and government agencies.
2. Expand Space Missions: Prioritize and fund upcoming space missions, such as the James Webb Space Telescope, the Europa Clipper, and Mars Sample Return missions. Ensure these missions include objectives related to the search for life and planetary formation studies.
3. Promote Collaboration: Facilitate international workshops and conferences to promote collaboration between scientists, engineers, ethicists, and policymakers. Create platforms for sharing data, technologies, and best practices.
Medium-Term Actions
1. Develop and Deploy Technologies: Advance the development of key technologies, such as high-resolution imaging systems and autonomous exploration vehicles. Begin deploying these technologies in space missions and experimental setups.
2. Establish Ethical Guidelines: Develop comprehensive ethical guidelines for space exploration, incorporating input from a diverse range of stakeholders. Ensure these guidelines are adopted by space agencies and private companies involved in exploration activities.
3. Enhance Public Outreach: Implement public outreach programs to educate and engage the public about space exploration, its potential benefits, and ethical considerations. Use social media, educational materials, and interactive exhibits to reach a broad audience.
Long-Term Actions
1. Sustain Research and Exploration: Maintain and expand research initiatives and space missions, continuously incorporating new technologies and findings. Foster a culture of innovation and interdisciplinary collaboration.
2. Implement Sustainable Colonization: Begin planning and implementing sustainable colonization efforts on the Moon, Mars, and potentially other celestial bodies. Ensure these efforts prioritize environmental stewardship and ethical considerations.
3. Monitor and Adapt: Regularly review and update ethical guidelines, technological strategies, and research priorities based on new discoveries and advancements. Remain adaptable to changing conditions and emerging challenges.
The recommendations and action plan outlined in this document provide a strategic roadmap for advancing our understanding of extraterrestrial life, planetary science, and the ethical implications of space exploration. By enhancing research and exploration capabilities, fostering technological innovation, and ensuring responsible stewardship, we can push the boundaries of human knowledge and explore the cosmos with integrity and foresight. The successful implementation of these strategies will require collaboration, creativity, and a commitment to ethical principles, guiding humanity toward a future where we can thrive both on Earth and beyond.
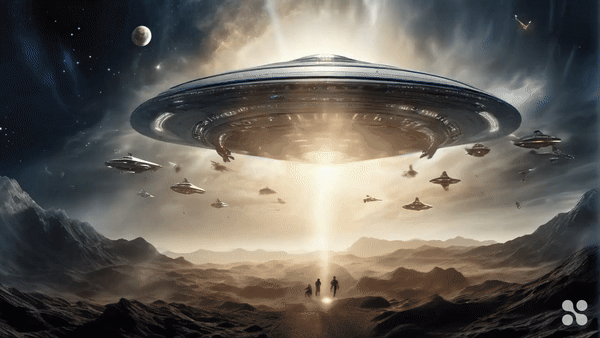
Section 15: Conclusion
Summary of Key Points
Geometric Patterns on Jupiter and Saturn
In this document, we have explored the intriguing geometric patterns found at the poles of Jupiter and Saturn. These patterns, including the octagonal and pentagonal cyclones on Jupiter and the hexagonal storm on Saturn, challenge our understanding of planetary atmospheres and suggest the possibility of intentional design by an advanced extraterrestrial civilization. Detailed studies and ongoing missions like Juno and Cassini have provided valuable data, revealing the complexity and stability of these structures and prompting new models of atmospheric dynamics.
Historical and Modern Perspectives on Alien Communication
The historical proposal by Carl Friedrich Gauss to use large geometric shapes as signals to potential extraterrestrial intelligences demonstrates the long-standing human fascination with communicating beyond our world. Modern interpretations of these ideas, combined with advancements in technology, offer new methods for signaling and detecting extraterrestrial life. Initiatives in radio and optical SETI continue to search for mathematical signals that could indicate intelligent life beyond Earth.
The Primer Concept and Its Implications
The hypothesis that the geometric patterns on Jupiter and Saturn serve as a primer from an advanced civilization invites us to consider the possibility of alien communication through universal mathematical principles. Building a geometric signal on Earth, such as the proposed Primer Beacon at the South Pole, represents a bold step in acknowledging and reciprocating potential extraterrestrial messages. This project involves significant technological and logistical challenges, but also promises profound scientific and cultural benefits.
Antarctic Colonization and the $PRIM Currency
The proposed Antarctic colony, supported by the $PRIM digital currency, aims to create a sustainable and vibrant community to facilitate the construction and maintenance of the Primer Beacon. This initiative addresses the logistical and environmental challenges of living in Antarctica, promoting renewable energy solutions and advanced technologies. By incentivizing participation and fostering a resilient economic system, $PRIM aims to make this ambitious project a reality.
Electromagnetic Considerations and Energy Harvesting
Understanding and mitigating the effects of strong magnetic fields on human health is crucial for the success of the Antarctic colony. Additionally, harnessing Antarctic magnetic fields and integrating renewable energy sources can provide a sustainable energy supply for the Primer Beacon and the colony. These efforts highlight the importance of advanced technology and interdisciplinary research in overcoming the challenges of this extreme environment.
Tesla's Wireless Energy Transmission
Building on Nikola Tesla's pioneering work, modern projects and technologies are exploring the feasibility of wireless energy transmission. By leveraging advanced materials and techniques, these systems aim to enhance energy accessibility and reduce environmental impact. The potential benefits of wireless energy transmission, including improved resilience and sustainability, align with the goals of the Primer Beacon project and broader efforts in space exploration.
Extraterrestrial Life and Genetic Parallels
The search for extraterrestrial life involves studying historical encounters, modern UFO sightings, and potential genetic parallels between Earth life and hypothetical alien life forms. Research in astrobiology and the study of extremophiles provides valuable insights into the resilience of life and the potential for its existence beyond Earth. Understanding the processes of planetary formation and habitability is crucial for identifying biosignatures and assessing the potential for life on exoplanets.
Terraforming Venus
Terraforming Venus represents a long-term vision for expanding human presence in the solar system. While current technology is not yet sufficient to undertake such an endeavor, ongoing research in astrobiology, extremophiles, and advanced materials continues to push the boundaries of what is possible. Ethical and environmental considerations are paramount in planning for the potential transformation of Venus into a more Earth-like world.
Astrobiology and Planetary Formation
Modern observations and discoveries of exoplanets have revolutionized our understanding of planetary formation. By studying Hot Jupiters and the dynamics of planetary systems, researchers are uncovering the processes that shape planets and their potential for supporting life. The search for biosignatures and the study of planetary habitability are central to the field of astrobiology, guiding future exploration and discovery.
Biblical Prophecies and Modern Science
The intersection of biblical prophecies and modern science offers a unique perspective on humanity's past, present, and future. By interpreting ancient texts through the lens of contemporary technology and scientific understanding, we can uncover new insights into our place in the universe. The potential discovery of extraterrestrial life and the expansion of humanity into space raise significant ethical and theological questions, inviting dialogue between science and religion.
Future Research Directions
The journey to understand our universe and our place within it is ongoing. Future research will continue to build on the foundations laid by past and current studies, exploring new frontiers in planetary science, astrobiology, and space exploration. Key areas for future research include:
1. Advanced Observational Techniques: Continued development of telescopes and instruments capable of detecting and analyzing exoplanet formation, and potential biosignatures.
2. Interdisciplinary Collaboration: Fostering collaboration between scientists, engineers, ethicists, and theologians to address the complex challenges and questions raised by space exploration and the search for extraterrestrial life.
3. Technological Innovation: Advancing technologies for renewable energy, wireless energy transmission, and autonomous systems to support sustainable exploration and habitation of extreme environments.
4. Ethical and Environmental Stewardship: Ensuring that the exploration and potential colonization of other planets are conducted responsibly, with careful consideration of ethical principles and environmental impacts.
The exploration of geometric patterns on Jupiter and Saturn, the search for extraterrestrial life, and the potential for space colonization represent some of the most profound and ambitious endeavors of humanity. By combining the insights of ancient texts with modern scientific research, we can expand our understanding of the universe and our place within it. As we continue to push the boundaries of knowledge and technology, the dialogue between science and religion, the integration of advanced technologies, and the commitment to ethical and environmental stewardship will be essential in guiding our journey into the cosmos.
Primer Research Patents
The Primer has filed the following essential energy production component patents:
1. Maxle: https://www.ic.gc.ca/opic-cipo/cpd/eng/patent/3123406/summary.html
2. YSolar: https://www.ic.gc.ca/opic-cipo/cpd/eng/patent/3115006/summary.html
3. Polar Beacon Generator: https://www.ic.gc.ca/opic-cipo/cpd/eng/patent/3108980/summary.html
Conversatons With ChatGPT
Checkmated ChatGPT on the classic dogma of conservation of energy in ~31 moves, must rewrite the texts (open to comments): https://docs.google.com/document/d/1iELKOE_AGdK2ho72ehvyzlHJx1cBSAsNgg2Qzw7Jvm0/edit?usp=sharing
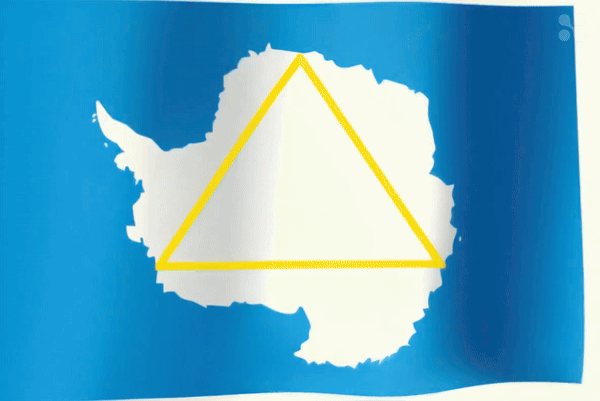